the Creative Commons Attribution 4.0 License.
the Creative Commons Attribution 4.0 License.
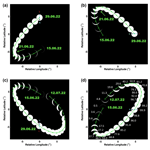
Earth observations from the Moon's surface: dependence on lunar libration
Nickolay Krotkov
Alexander Marshak
Observing the Earth from the Moon's surface has important scientific advantages. The angular diameter of the Earth as seen from the Moon's surface is 1.8–2.0∘ (the angular size varies due to the change in the Earth–Moon distance). The libration of the Moon in latitude reaches an amplitude of 6.68∘ and has a main period of 27.21 d (or 653.1 h). The libration of the Moon in longitude, reaching an amplitude of 7.9∘, has a period of 27.55 d (or 661.3 h). This causes the center of the Earth to move in the Moon's sky in a rectangle measuring 13.4∘ × 15.8∘. The trajectory of the Earth's motion in this rectangle changes its shape within a period of 6 years. This apparent librational movement of the Earth in the Moon's sky complicates observations of the Earth. This paper proposes that this disadvantage be turned into an advantage and that a multi-slit spectrometer be placed on the Moon's surface on a fixed platform. The libration motion and the daily rotation of the Earth will act as a natural replacement for the scanning mechanism.
- Article
(7945 KB) - Full-text XML
- BibTeX
- EndNote
The scientific benefits of observations from the Moon for the Earth, exoplanet, and astrophysics studies are discussed in several recent papers (Marshak et al., 2020; Gorkavyi et al., 2021; Boyd et al., 2022). Although current Earth-observing satellites can produce high-resolution images, low Earth orbit (LEO) sensors can only scan a small portion of the globe at a given time, while geosynchronous equatorial orbit (GEO) sensors can provide temporally continuous, though lower-resolution, observations of a significant, but fixed, portion of the Earth's disk. The Earth Polychromatic Imaging Camera (EPIC) on the Deep Space Climate Observatory (DSCOVR) clearly stands apart, observing the entire Sun-illuminated Earth from the L1 Sun–Earth Lagrange point (Marshak et al., 2018). The L1 location, however, limits phase (i.e., Sun–Earth–camera) angles to a nearly backscattering direction (up to ∼ 178∘). The phase angle interval from 2 to 12∘ is determined by the trajectory of the DSCOVR space observatory, which does not rest at the Lagrange point but moves around it. It is too “noisy” to transmit data directly from the Earth–Sun line; thus, the phase angle is bigger than 2∘. A compact, lightweight, autonomous camera and a spectrometer on the Moon's surface offer a unique opportunity to complement these observations and to image the full range of Earth's phases, potentially advancing Earth science in many ways (Marshak et al., 2020; Gorkavyi et al., 2021) – some examples are listed as follows:
-
observing ocean–cloud glints at different phase angles;
-
comprehensive whole-globe monitoring of transient volcanic and aerosol clouds, including the strategically important (for climate studies) polar regions not covered by GEO;
-
detection of polar mesospheric and stratospheric clouds;
-
estimating the bidirectional surface reflectance factor (BRF) and full phase-angle-integrated albedo;
-
monitoring and quantifying changes in vegetation;
-
simultaneous imaging of the day and night parts (i.e., the twilight zone) during crescent phases of the Earth and shadowed parts illuminated by the Moon.
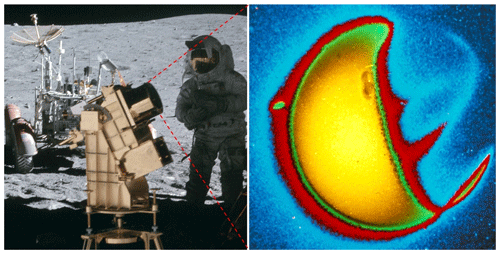
Figure 1Left: a far-ultraviolet camera and spectrograph was operated on the lunar surface during the Apollo 16 mission, April 1972 (credits: NASA, Apollo 16). Right: the Earth, photographed in far-ultraviolet light (1304 angstroms) by astronaut John W. Young. Credits: George Carruthers (NRL) and Thornton Page (JSC), Far UV Camera, NASA, Apollo 16; based on the image AS16-123-19657 (Mason, 2019).
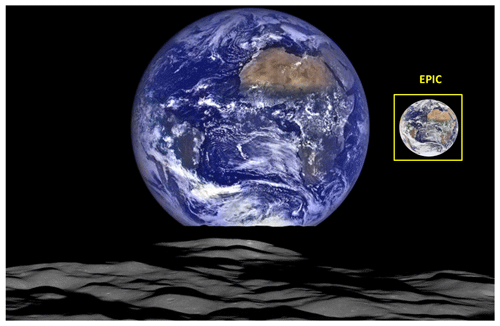
Figure 2A unique view of Earth from the LRO's vantage point in orbit around the Moon (12 October 2015). LRO was about 134 km above the Moon's far-side crater Compton (55∘ N, 104∘ E). The photograph is a combination of images in seven color bands from a wide-angle camera (WAC) and black and white images from two narrow-angle linear push-broom cameras (NACs) with a linear (one-dimensional) array of 5064 elements. Each NAC camera has a field of view of 2.86∘. Image credit: NASA, GSFC, Arizona State University (https://www.nasa.gov/image-feature/goddard/lro-earthrise-2015, last access: 18 March 2023). LRO data (Burns et al., 2012; Keller et al., 2016) can be of great help in planning Earth observations from the lunar surface. Insert shows an image of the same part of the Earth taken by DSCOVR-EPIC on the same day (12 October 2015) as it would be seen from the Lagrange point.
The first telescopic image of the Earth from the Moon was obtained during the expedition of Apollo 16 in 1972 using an ultraviolet telescope (Carruthers and Page, 1972) – see Fig. 1. In later years, prospects for lunar observations of the Earth have been discussed in many papers (e.g., Foing, 1996; Moccia and Renga, 2010). Observations of the Earth with instruments mounted on the Moon have been actively discussed in recent years (Hamill, 2016). Impressive prospects for observing the Earth from the Moon in the visible spectrum are demonstrated in the pictures taken by the Lunar Reconnaissance Orbiter (LRO) in 2015. The difference in distances (Earth–Moon and Earth–L1) leads to the fact that a telescope with the same field of view sees the Earth with different angular sizes and resolutions (Fig. 2).
One of the objectives of the Chinese space program is Moon-based observation of the Earth (Li et al., 2019; Guo et al, 2019). A lunar lander, Chang'e-3 (which landed on the Moon in 2014 and is still working), is equipped with a 5 cm ultraviolet telescope and extreme UV camera and studies changes in the Earth's plasmasphere in the UV range (He et al., 2016).
One of the tasks facing the US Artemis program is as follows:
Use the Moon as a platform for Earth-observing studies … The observations from the Moon will have higher resolution than would similar observations made from L1. Myriad science investigations targeting topics such as lightning, Earth's albedo, atmosphere, and exosphere …, the oceans, infrared emission, and radar interferometry may be accomplished from the surface of the Moon. The Moon also offers a unique vantage point for full-disk observations … (Artemis III Science, 2020).
Because of tidal locking, the Moon's rotation around its axis is synchronized with its orbital rotation around the Earth. Therefore, the Moon always faces the Earth on one side, and the task of observing the Earth from the Moon seems simple: the Earth must hang motionless in the lunar sky, rotating around its axis. In reality, the Earth moves along a complex trajectory in the sky of the Moon due to lunar librations in latitude and longitude. On the one hand, the librations of the Moon cause the Earth to shift from the field of view of the lunar telescope, which forces one to turn the telescope to track the Earth's observable movement in the Moon's sky (guiding); on the other hand, for a fixed-slit spectrometer, the librations of the Moon can be useful because they make the Earth move through the fixed field of view of the instrument (across the slit). These librations can serve as a natural mechanism for scanning the Earth when observed from the Moon, which allows for the use of slit spectrometers and telescopes on a fixed platform. This paper takes into account lunar librations and analyzes the conditions for observing the Earth from the Moon.
Observations of the Earth from the Moon require accounting for the geometry of the relative position of the centers of the Earth and the Moon, the inclinations of their axes, and the libration effects (Meeus, 1991, 2000; Guo et al., 2018; Xu and Chen, 2019; Huang et al., 2020).
Libration of the Moon in latitude. The angle between the Moon's axis of rotation (N–S or north–south) and the normal to the plane of its orbit around Earth (PP′) is 6.68∘ (Fig. 3). This causes the libration of the Moon in latitude with the same amplitude and with a period of draconic month TD = 27.21222 d or 653.0933 h (the interval between consecutive passages of the Moon through the same node of the orbit; an orbital node is either of the two points where a lunar orbit intersects an ecliptic plane to which it is inclined) – see, for example, Meeus (1991, 2000). As a result of this inclination, parts of the Moon's polar regions are accessible for observations from the Earth.
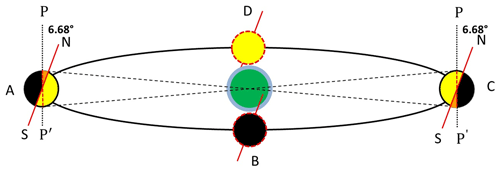
Figure 3Libration in latitude results from an inclination of 6.68∘ between the Moon's axis of rotation (NS) and the normal to the plane of its orbit around Earth (PP′). The hemisphere of the Moon that is visible from Earth at point D is marked in yellow; black is the invisible hemisphere at point B. Additional areas of the lunar surface that become available for observation at points A and C are marked in orange. View from the point close to the ecliptic plane.
Libration of the Moon in longitude. The Moon moves around the Earth in an elliptical orbit with an average eccentricity (or deviation of an orbit from circularity) e=0.055 (it varies between 0.0255 and 0.0775) and a period of anomalistic month TA = 27.55455 d or 661.3092 h – the interval between consecutive passages of the Moon through the perigee or the apogee of its orbit, where a is the semi-major axis a = 384 399 km (Fig. 4). This causes libration in longitude with an amplitude of 7.9∘ (see, for example, Meeus (1991, 2000)).
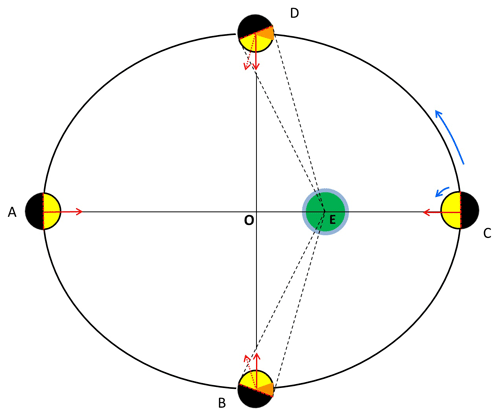
Figure 4Libration in longitude results from the eccentricity of the Moon's orbit. It can reach 7.9∘ in amplitude. Point A is the apogee of the lunar orbit (AE = rmax); point C is the perigee (EC = rmin); AO = a; OE = ae; points B and D are co-vertices; the semi-minor axis OB = OD = . Additional areas of the lunar surface that become available for observation are marked in orange. View from the North Pole of the Earth.
The longitudinal libration consists of two components:
-
An ellipse with a small eccentricity (in the first approximation) can be described as a circle around the Earth, which is shifted from the center of the circle (point O) by the distance OE = ea (see Fig. 4). This displacement leads to the fact that the observer from the Earth begins to see part of the lateral surfaces of the Moon (Fig. 4).
-
If the Moon was moving along an orbit at a uniform speed, then its visible part would always be directed to the center of the orbit (point O in Fig. 4). But the speed of the moon changes due to the ellipticity of the orbit. If at perigee the Moon is turned to the Earth as in Fig. 4 at point C, then in a quarter of the anomalistic month , it should turn 90∘ counterclockwise at point D (see the solid red arrow at point D). But due to the high velocity along the orbit segment CD, the Moon arrives at point D faster than , so the Moon does not have time to turn 90∘ (see the dashed red arrow at point D). This further increases the area of the Moon's surface that is visible from Earth. On segment DA, the Moon's orbital speed slows down, and the Moon has time to turn 180∘ at point A. With the slow motion of the Moon along segment AB, the Moon has time to rotate around its axis by more than 90∘, which again increases the surface area available for observations from the Earth. On segment BC, the Moon's orbital velocity increases: the Moon passes through the BC segment faster than , so it does not have time to turn 90∘, and as a result of this lag, the Moon returns to its initial position at point C.
Figure 5 shows the librations of the Moon in longitude and latitude for 2022 (Espenak, 2021). The selenographic coordinate system repeats the Earth's; therefore, the selenographic center of the Moon's disk is the intersection point of the lunar equator and the lunar prime meridian. The selenographic zero corresponds to the average position of the center of the visible disk of the Moon. At any particular moment in time, the center of the visible disk of the Moon can shift from the selenographic zero due to libration. If the apparent center shifts along the lunar equator, then we call this shift the relative longitude of the libration; if it shifts along the meridian, then we call this shift the relative latitude of the libration. In other words, latitude and longitude libration (or relative libration) is the visual displacement of the selenographic center of the Moon's disk (0∘ lunar latitude and 0∘ lunar longitude) relative to the center of the visible disk of the Moon. Libration in longitude correlates with variations in the Earth–Moon distance and changes more strongly with time than libration in latitude.
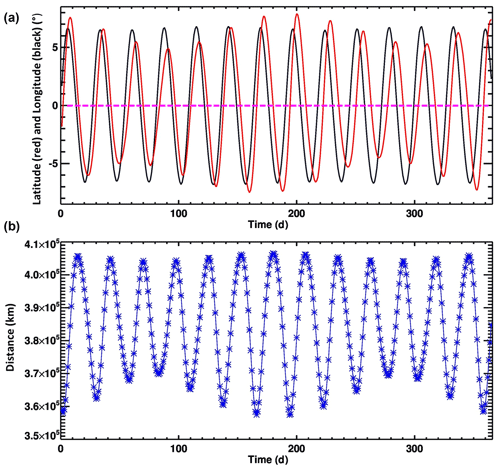
Figure 5Variability of the Moon's orientation and orbit during 2022, starting from 1 January 2022 (Espenak, 2021). The distance between the Earth and the Moon is measured between the centers of the bodies, so it does not depend on libration, which is measured in angles relative to the centers of the bodies. (a) Longitude libration (red) and latitude libration (black). The straight dashed line corresponds to the case of zero libration – that is, when the center of the visible lunar disk coincides with the zero point of selenographic longitude and latitude. (b) The Earth–Moon distance. The time-averaged distance between the centers of Earth and the Moon is 385 000 km; the minimal distance is 356 500 km, and the maximal distance is 406 700 km.
The libration of the Moon discussed above (Figs. 3 and 4) is called optical libration. The tidal action of the Earth causes physical libration associated with a change in the period of the Moon's own rotation. Physical libration is only 2 arcmin – that is, much less than optical libration. In the calculations (Fig. 5), physical libration is taken into account along with optical libration.
Obviously, the discussed librations of the Moon are directly related to the observation point on the Moon's surface: if the observation point on the Moon changes its angle relative to the Earth–Moon line, then the Earth also changes its position in the lunar sky by the same amount but the different sign (in an approximation where the size of the Moon can be neglected compared to the Moon–Earth distance). In other words, if the lunar telescope raises its line of sight by +5∘ above the line connecting the centers of the Earth and the Moon, then the Earth goes down from the telescope's line of sight by −5∘.
An observer can see the Earth from any point in the Moon's visible hemisphere (Fig. 6). The location of the observer will affect (i) the position of the zero point of Earth's libration in latitude and longitude and (ii) the orientation of the trajectory of the apparent libration of the Earth in the sky of the Moon.
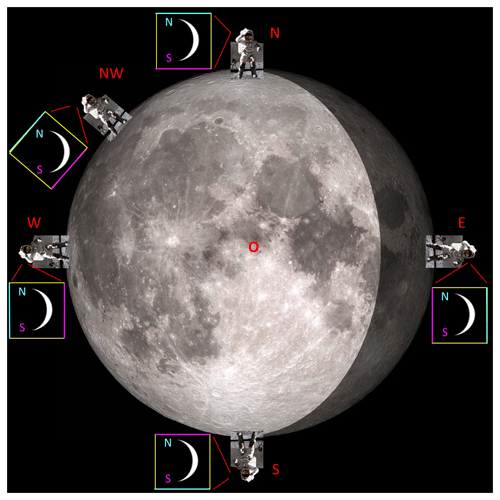
Figure 6Observers in different points of the Moon's visible hemisphere. Image of the Moon – LRO (NASA, GSFC, Arizona State University) https://www.nasa.gov/feature/goddard/2020/moon-more-metallic-than-thought (last access: 18 March 2023). Observer: photo of John W. Young, commander of the Apollo 16 lunar-landing mission (NASA). It is shown how, from the point of view of different observers on the Moon, the crescent of the Earth is oriented, indicating the Earth's poles. The yellow squares (with blue top and violet bottom lines) show the astronaut's vertically oriented field of view (FOV) and the crescent of the Earth that they see in this FOV (or in frame of the camera).
From the point of view of an Earth observer, lunar librations in latitude and longitude are measured as a relative displacement from the lunar zero longitude and longitude – that is, from the point of the lunar disk taken as the zero point and located in the center of the visible disk of the Moon, near the crater Möstig A. From the point of view of a lunar observer located near this crater at the intersection of the lunar equator and the lunar zero meridian (see point O in Fig. 6), the Earth hangs above a given point on the lunar surface (at the zenith). Therefore, if the observer moves away from this point along the lunar meridian, for example, to the lunar north pole (point N in Fig. 6), then the apparent position of the center of the Earth will also shift, moving to the horizon. When the observer is at the lunar pole, the Earth will hang on the horizon. If the observer goes again to the equator and not along the zero meridian but rather along the meridian with a longitude of 90∘ (for example, to the west; see points NW and W in Fig. 6) – that is, along the border between the visible and invisible hemispheres of the Moon, then the Earth will remain hanging above the lunar horizon but will change the apparent tilt of its axis of rotation. For an observer at the Moon's equator (points W, O, E in Fig. 6), the Earth's axis will tilt 90∘ – that is, the Earth will “lay on its side”.
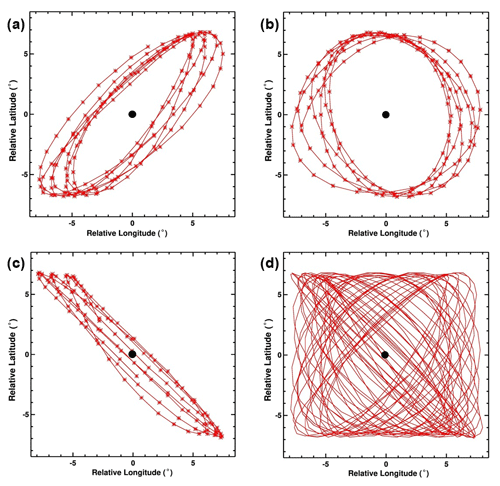
Figure 7Visual librations of Earth in the Moon's sky during (a) the first 6 months 2022, (b) the first 6 months 2023, (c) July–October 2024, and (d) 2022–2024 (the dots are deleted). The latitude and longitude of the libration of the Moon (Giesen, 2018; Espenak, 2021) were converted to the relative Earth libration angles using a sign change. The positions of the Earth in the sky of the Moon are plotted in increments of a day. If there were no lunar libration, then the Earth would be at the black dot in the center of the figures.
When the observer reaches the south pole (point S in Fig. 6), the Earth will be turned 180∘ relative to it. Similar changes will occur with the trajectories of the Earth in the sky of the Moon. Libration of the Moon sets the trajectory of the Earth in the lunar sky described by relative latitude and longitude (relative to the point of zero libration, marked with a black dot in Fig. 7). The shape of this trajectory (see the red trajectories in Fig. 7) is strictly defined and does not depend on the position of the observer on the Moon's surface. But the height of the point of zero libration above the horizon depends on the position of the lunar observer, as well as the orientation of the libration trajectory – that is, the rotation of the visible libration trajectory around this point of zero libration. An analogy is a picture hanging on the wall of a room. The pattern in the picture does not depend on the position of the observer, but they can stand on their head and completely change the orientation of the pattern relative to their field of vision.
We can take the latitude and longitude of the libration of the Moon (Giesen, 2018; Espenak, 2021; Fig. 5) and plot the positions of the Earth in the sky of the Moon for each day (Fig. 7). Each dot in the Fig. 7a, b, and c represents the latitude and longitude for a particular day.
Figure 7a shows the visual position of the Earth for the first half of 2022. Figure 7b shows the apparent libration of the Earth for the first half of 2023, and Fig. 7c shows the apparent libration for 4 months (July–September) of 2024. Figure 7d shows the trajectory of the Earth in the sky of the Moon for 3 years (2022–2024). The orientation of the libration pattern in Fig. 7 corresponds to the position of the observer on the line of the zero-meridian S–O. At point O, the zero-libration point is above the observer's head, and when the observer moves to the south pole (point S), the zero-libration point shifts to the horizon.
It can be seen that the shapes of the curves along which the Earth moves in the sky of the Moon change noticeably over 3 years.
The movement of the Moon around the Earth can be characterized by three periods:
-
draconic month TD = 27.21222 d or 653.0933 h (the period of movement relative to the ascending or descending node)
-
anomalistic month TA = 27.55455 d or 661.3092 h (the period of movement relative to the perigee)
-
sidereal month TS = 27.32166 d or 655.7198 h (the period of movement relative to the stars).
A beat is an interference pattern between two slightly different frequencies, perceived as a periodic variation in amplitude whose rate is the difference of the two frequencies. As a result of three slightly different lunar periods, we have three different beats or precession frequencies.
The apsidal precession period is TSA=8.85 years and is found by the following formula:
The nodal precession period is TDS=18.6 years and is found by the following formula:
The librations of the Moon in latitude and longitude follow a 6-year cycle, when the major axis of the lunar orbit has performed one complete revolution with respect to the line of nodes (Meeus, 1991, 2000; Giesen, 2018):
with a period of TDA=2190.34 d or 6 (more precisely, 5.99667) anomalistic years (365.259636 d each). All three periods of precessions are connected:
Figure 8a shows the position of the center of the Earth in the lunar sky for 6 years (2022–2027). Figure 8b shows the statistics of the distribution of the 2191 positions of the center of the Earth for this period. The average distribution density of the center of the Earth in squares 1∘ × 1∘ (or the number of entries of the center of the Earth into this square for 6 years) is = = 8.6 per pixel; in reality N ranges from 0 to 34.
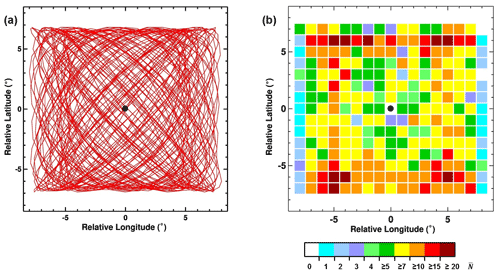
Figure 8Visual libration of Earth during 2022–2027 (6 years or 2191 d): (a) trajectory of the center of the Earth in the sky of the Moon; (b) statistics of the distribution of the 2191 positions of the center of the Earth for 6 years. is the number of entries of the Earth center into each 1∘ × 1∘ grid cell for this period (or how many days the Earth spent in a 1∘ × 1∘ pixel).
Spectrometric observations through the slit are a common practice for many satellite observations of the Earth. Scanning of the Earth's surface is usually carried out by movement of the low orbit satellites. For observation from the Moon, it is logical to consider the option when scanning occurs due to the libration and diurnal motion of the Earth. The scientific goals for the slit observation are close in both cases.
The angular velocity of a point on the Earth's surface in the field of view of the sensor is caused by two comparable factors: the rotation of the Earth around its axis and lunar libration, which causes a shift in the center of the Earth. The rotation of the Earth around its axis is a well-studied process, but librations of the center of the Earth in the lunar sky are poorly understood and raise many questions. When observing the Earth through the slit of the spectrometer, it will be necessary to take into account both the displacement of the center of the Earth and the Earth's rotation.
The librational apparent motion of the Earth must be taken into account when observing from the Moon and can also become a natural substitute for scanning (Fig. 9). It is proposed that fixed-mount instruments be installed on the Moon's surface, directed towards the Earth.
-
A hyperspectral sensor (UV, Vis, NIR, IR) will observe Earth passing through fixed vertical slits. The librations of the Moon and the daily rotation of the Earth will serve as a natural scanning mechanism for this spectrometer. This multi-slit spectrometer can be similar to the six-slit hyperspectral limb profiler (LP) on the OMPS aboard Suomi National Polar-orbiting Partnership (S-NPP) LEO satellite, as well as the single-slit hyperspectral Ozone Monitoring Instrument (OMI) on the NASA Earth Observing System (EOS) Aura satellite. Each LP slit uses approximately of the detector matrix. A multi-slit spectrometer for observing the Earth from the surface of the Moon can have six to eight slits, whose fields of view are shifted by 2.5∘. Since the maximum angular size of the Earth is 2∘, the angular distance between the lines of sight of neighboring slits must be greater than the angular diameter of the Earth so that light from the Earth does not hit two slits at the same time. Each slit can use the entire matrix because they scan the Earth at different times in turn and do not interfere with each other.
-
A wide-field-of-view (WFOV) ∼ 18–20∘ camera will continuously image the Earth in any points of trajectory, including a part of the lunar surface with a true-color calibration target. The camera can be hyperspectral, with the inclusion of wavelengths that EPIC uses.
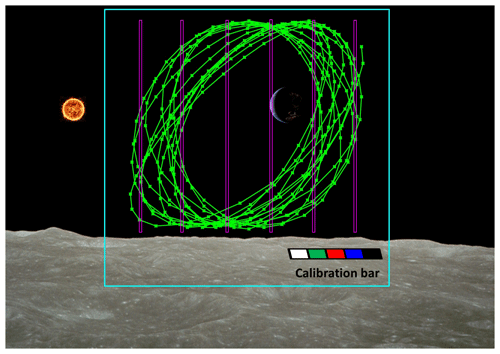
Figure 9Visual positions of the Earth's center during 2026 (green line) from the south pole of the Moon (point S in Fig. 6) and possible positions of slits of the spectrometer (violet). The blue square is ∼ 18–20∘ FOV of fixed-mount camera. The calibration bar is used to calibrate color images from a wide-angle camera. Lunar surface is from the photo taken by NASA (Apollo).
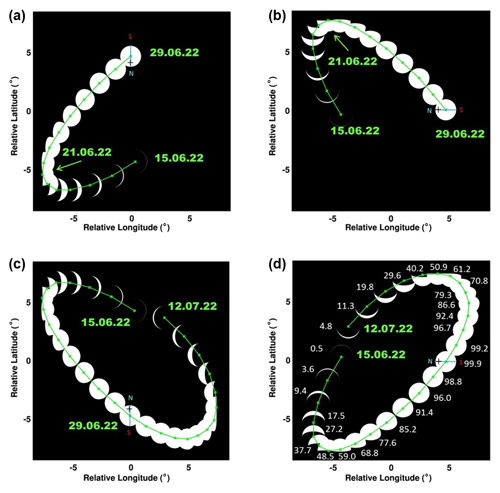
Figure 10Positions of the Earth for different Moon observers. (a) Position of the Earth's center and Earth's phases during 15–29 June 2022 (15 points, green line) for an observer located on the lunar south pole (the point S in Fig. 6). The Earth is almost completely illuminated on 29 June 2022, while its axis is tilted to the Sun at an almost maximum angle (summer in the Northern Hemisphere), which creates good conditions for observing the Earth's North Pole (marked with a cross and the letter N). (b) Earth's phases and visible trajectory for the same period for an observer on the equator near longitude 90∘ W (the point W in Fig. 6). (c) Position of the Earth center and Earth's phases during 15 June–12 July 2022 (28 points, green line) for an observer located on the lunar north pole (the point N in Fig. 6). (d) Earth's phases and visible trajectory for the same period for an observer on the equator near longitude 90∘ E (the point E in Fig. 6).
It should be noted that the longitude of the observation point on the Moon affects the orientation of the visible trajectory of the Earth in the sky of the Moon. For example, the diagonally elongated trajectory of the Earth for July–October 2024 (Fig. 7c, for the case of lunar longitudes near 0∘ and for the observer at point S in Fig. 6) will have a different orientation when observed from the zone of lunar longitudes of about 45∘ N for the observer at point NW in Fig. 6. The orientation of the Earth's libration trajectories in the sky of the Moon will change accordingly (see Fig. 10). The sun in the region of the lunar poles moves almost parallel to the horizon, and in the region of the lunar equator, it passes through the zenith, descending vertically to the horizon or rising from it.
Figure 6 shows the orientation of the Earth's crescent from the point of view of different observers on the Moon and helps interpret the orientation of the libration pattern in Fig. 10.
The portion of the illuminated Earth does not change with the position of the Moon's observer, but it changes during the lunar month (Fig. 10, based on data by Espenak, 2021).
These arguments must be taken into account when planning observations of the Earth from the Moon (or when communicating between the Moon and the Earth). Satellites located at the Earth–Moon Lagrange points will move along similar trajectories in the sky of the Moon.
The principal design of the multi-slit spectrometer is shown in Fig. 11. Its main feature is that it uses only one matrix detector for many slits. This is due to the fact that a local object such as the Earth can pass only one slit at a given moment. Therefore, it is possible to image the light from all slits onto a single matrix without compromising observations, although the problem of scattered light may exist and should be studied in the development of a specific instrument. Each slit is directed to a unique position of the Earth in the Moon's sky, but the spectral range of all slits is the same. If the spectra are taken with a slit that occupies a length of 4000 pixels on the detector matrix, then the spectra will be determined from the part of the Earth with a size of ∼ 30 km along the slit. The effective width of this pixel across the slit (i.e., spatial resolution) will depend on the frequency of observations, the width of the slit, and the velocity of the Earth moving across the slit.
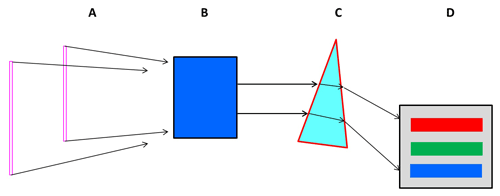
Figure 11The principal design of a spectrometer that has multiple slits (A), a collimator (B), a prism (C), and a single two-dimensional detector array (matrix D). The spectrometer merges the light from all the slits together, but since the Earth always occupies only one slit, the signals from different slits do not interfere with each other.
The angular diameter of the Earth in the sky of the Moon is 1.8–2.0∘. The typical rate of displacement of the center of the Earth is 1–2∘ per day (see Figs. 7, 10). Therefore, the passage of the Earth through each individual slit of the spectrometer will take 1–2 d. During this time, the Earth makes one to two rotations around its axis, which will allow each slit to receive at least one scan of the entire Earth's surface in one pass. The potential scan frequency depends on the field of view of the device and on the detector matrix used so that the spatial pixel across the slit is comparable to the size of the spatial pixel along the slit. For a detector matrix with a size of 1000–4000 pixels and a field of view of 5 to 15∘, the scan frequency should be 10–100 s.
Important Earth science goals for such a spectrometer are to complement and improve the current DSCOVR-EPIC whole-Earth imaging (Gorkavyi et al., 2021). The acquired data will enable the estimation of aerosol and cloud scattering phase functions, the amount of trace gases, and the surface bidirectional reflectance factor (BRDF).
-
Due to lunar libration, the center of the Earth for an observer on the Moon moves in a rectangular area with dimensions of 13.4∘ × 15.8∘. The density of the location of the Earth in this rectangular area is an average of 8.6 per square degree over 6 years (2191 d). The density for different parts of the area varies from 0 to 34.
-
The movement of the Earth in the sky of the Moon is characterized by quasi-periodicity with frequencies of ∼ 27 d and 6 years. The rates of displacement of the Earth in the Moon's sky reach 2∘ per day. The shape of the Earth's trajectory changes from a circle to a straight line (see Fig. 7).
-
Lunar libration must be taken into account when observing the Earth from the surface of the Moon and during Moon–Earth communications.
This paper discusses Earth observations from the Moon's surface, both spectroscopically and in the imaging mode. The librations of the Moon in the range of 13–16∘ and the daily rotation of the Earth serve as a natural scanning (or guiding) mechanism for a spectrometer with vertical slits. This greatly simplifies the design of the spectrometer. We suggest a lightweight EPIC-Moon instrument on a fixed platform to serve as a proof of concept for Earth observations, as well as for the whole Earth true-color imagery to the public.
The lunar environment may present serious problems for the operation of sensors due to dust settling and impact on moving parts and due to the influence of high-energy particles and meteoroids. The discussed design of instruments on a fixed mount, with no movement of the external parts of the instruments, significantly reduces the dependence of observations on lunar dust. During the use of such instruments on the lunar surface, the rate of dust settling and the degree of degradation of instruments due to radiation will be clarified, which will make it possible to optimize the design of future instruments and to protect them as much as possible from a hostile environment.
There is a particular interest in observations of the lunar dust environment during total solar and lunar eclipses (which happen ∼ two times a year when the Earth blocks the Sun): the lunar dust glows from scattered sunlight under forward-scattering conditions without saturation of the detector.
The proximity to the Earth (versus the L1 point) and wide variations in phase angle accessible by a Moon-based camera offer unique advantages for observations of the bidirectional land surface reflectance; ocean–cloud glint reflection; whole-globe monitoring of transient volcanic and/or aerosol clouds, as well as polar mesospheric and stratospheric clouds; vegetation; and the twilight zone and shadowed parts of the Earth illuminated by the Moon.
Planetary Ephemeris Data Courtesy of Fred Espenak. Data are available at http://www.astropixels.com/ephemeris/ephemeris.html (Espenak, 2021).
NG developed computer codes and algorithms, analyzed the results, and wrote the paper. NK and AM participated in the algorithm development, analyzing the results, and writing the paper.
The contact author has declared that none of the authors has any competing interests.
Publisher’s note: Copernicus Publications remains neutral with regard to jurisdictional claims in published maps and institutional affiliations.
The authors thank the Apollo, LRO, and EPIC-DSCOVR teams for providing the data presented. The authors are grateful to Fred Espenak for the useful Planetary Ephemeris data. We also thank Padi Boyd and our colleagues from Goddard Space Flight Center interested in the Earth observations from the Moon's surface.
Nickolay Krotkov and Alexander Marshak were supported by the NASA DSCOVR project managed by Richard Eckman. Alexander Marshak was supported by the Goddard Artemis project managed by Michele Gates. Nick Gorkavyi was partially supported by the NASA Aura project (OMI core team) managed by Ken Jucks.
This research has been supported by the Goddard Space Flight Center (Aura project (OMI core team), Artemis project, and DSCOVR project).
This paper was edited by Jun Wang and reviewed by Liviu Ivanescu and two anonymous referees.
Artemis III Science: Definition Team Report, NASA/SP-20205009602, https://www.nasa.gov/sites/default/files/atoms/files/artemis-iii-science-definition-report-12042020c.pdf (last access: 19 March 2023), 2020.
Boyd, P. T., Wilson, E. L., Smale, A. P., Supsinskas, P., Livengood, T. A., Hewagama, T., Villanueva, G. L., Marshak, A., Krotkov, N. A., Pokorny, P., Bixler, J. V., Noland, J. D., Ramu, G., Cleveland, P., Ganino, J., Jhabvala, M. D., Quintana, E. V., Gilbert, E., Colón, K. D., Arney, G. N., Domagal-Goldman, S. D., Mandell, A. M., Barclay, T., Kuchner, M. J., and Ott, L.: EarthShine: Observing our world as an exoplanet from the surface of the Moon, Journal of Astronomical Telescopes, Instruments, and Systems, 8, 014003, https://doi.org/10.1117/1.JATIS.8.1.014003, 2022.
Burns, K. N., Speyerer, E. J., Robinson, M. S., Tran, T., Rosiek, M. R., Archinal, B. A., Howington-Kraus, E., and the LROC Science Team: DIGITAL ELEVATION MODELS AND DERIVED PRODUCTS FROM LROC NAC STEREO OBSERVATIONS, Int. Arch. Photogramm. Remote Sens. Spatial Inf. Sci., XXXIX-B4, 483–488, https://doi.org/10.5194/isprsarchives-XXXIX-B4-483-2012, 2012.
Carruthers, G. R. and Page, T.: Apollo 16 Far-Ultraviolet Camera/Spectrograph: Earth Observations, Science, 177, 788–791, https://doi.org/10.1126/science.177.4051.788, 1972.
Espenak, F.: Planetary Ephemeris Data, Astropixels Publishing [data set], http://www.astropixels.com/ephemeris/ephemeris.html (last access: 19 March 2023), 2021.
Foing, B. H.: The Moon as a platform for astronomy and space science, Adv. Space Res., 18, 1117–1123, 1996.
Giesen, J.: Moon Libration Applet, http://www.jgiesen.de/moonlibration/ (last access: 19 March 2023), 2018.
Gorkavyi, N., Krotkov, N., Gorkavyi, N., Marchenko, S., Vasilkov, A., Knyazikhin, Y., Kowalewski, M., Torres, O., DeLand, M., Ramsey, M., Christensen, P., and Realmuto, V.: Earth Imaging From the Surface of the Moon With a DSCOVR/EPIC-Type Camera, Front. Remote Sens., 2, 724074, https://doi.org/10.3389/frsen.2021.724074, 2021.
Guo, H., Liu, G., and Ding, Y.: Moon-based Earth observation: scientific concept and potential applications, Int. J. Digit. Earth, 11, 546–557, https://doi.org/10.1080/17538947.2017.1356879, 2018.
Guo, H., Fu, W., and Liu, G.: Scientific Satellite and Moon-Based Earth Observation for Global Change, Springer, Singapore, https://doi.org/10.1007/978-981-13-8031-0, 2019.
Hamill, P.: Atmospheric observations from the moon: A lunar earth-observatory, IGARSS 2016 – 2016 IEEE International Geoscience and Remote Sensing Symposium, 10–15 July 2016, Beijing, China, https://doi.org/10.1109/IGARSS.2016.7729964, 2016.
He, H., Shen, C., Wang, H., Zhang, X., Chen, B., Yan, J., Zou, Y., Jorgensen, A. M., He, F., Yan, Y., Zhu, X., Huang Y., and Xu, R.: Response of plasmaspheric configuration to substorms revealed by Chang'e 3”, Sci. Rep., 6, 32362, https://doi.org/10.1038/srep32362, 2016.
Huang, J., Guo, H., Liu, G., Shen, G., Ye, H., Deng, Y., and Dong, R.: Spatio-Temporal Characteristics for Moon-Based Earth Observations, Remote Sens., 12, 2848, https://doi.org/10.3390/rs12172848, 2020.
Keller, J., Petro, N., Vondrak, R. and the LRO team: The Lunar Reconnaissance Orbiter Mission – Six years of science and exploration at the Moon, Icarus, 273, 2–24, https://doi.org/10.1016/j.icarus.2015.11.024, 2016.
Li, C., Wang, C., Weiand, Y., and Lin, Y.: China's present and future lunar exploration program, Science, 365, 238–239, https://doi.org/10.1126/science.aax9908, 2019.
Marshak, A., Herman, J., Szabo, A., Blank, K., Carn, S., Cede, A., Geogdzhaev, I., Huang, D., Huang, L.-K., Knyazikhin, Y., Kowalewski, M., Krotkov, N., Lyapustin, A., McPeters, R., Meyer, K., Torres, O., and Yang, Y.: Earth Observations from DSCOVR/EPIC Instrument, B. Am. Meteorol. Soc., 9, 1829–1850, https://doi.org/10.1175/BAMS-D-17-0223.1, 2018.
Marshak, A., Krotkov, N., Gorkavyi, N., Marchenko, S., Vasilkov, A., Knyazikhin, Y., Kowalewski, M., Torres, O., DeLand, M., Ramsey, M., Christensen, P., and Realmuto, V.: Whole Earth imaging from the Moon South Pole (EPIC-Moon), WhitePaper # 2054 in Artemis III Science Def. Team Report, 2020.
Mason, T.: Earth's Shining Upper Atmosphere – From the Apollo Era to the Present, University of Central Florida, Orlando, FL, https://gold.cs.ucf.edu/earths-shining-upper-atmosphere-from-the-apollo-era-to-the-present/ (last access: 18 March 2023), 2019.
Meeus, J.: Astronomical Algorithms, 1st English edn., Willmann-Bell, Richmond, Virginia, ISBN 978-0943396354, 1991.
Meeus, J.: Mathematical Astronomy Morsels, 2nd edn., Willmann-Bell, Richmond, Virginia, ISBN 978-0943396514, 2000.
Moccia, A. and Renga, A.: Synthetic Aperture Radar for Earth Observation from a Lunar Base: Performance and Potential Applications, IEEE T. Aero. Elec. Sys., 46, 1034–1051, https://doi.org/10.1109/TAES.2010.5545172, 2010.
Xu, Z. and Chen, K.-S.: Effects of the Earth's Curvature and Lunar Revolution on the Imaging Performance of the Moon-Based Synthetic Aperture Radar, IEEE T. Geosci. Remote, 57, 5868–5882, https://doi.org/10.1109/TGRS.2019.2902842, 2019.