the Creative Commons Attribution 4.0 License.
the Creative Commons Attribution 4.0 License.
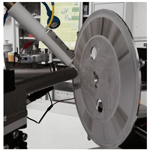
STRAS: a new high-time-resolution aerosol sampler for particle-induced X-ray emission (PIXE) analysis
Silvia Nava
Roberta Vecchi
Paolo Prati
Vera Bernardoni
Laura Cadeo
Luca Carraresi
Carlo Cialdai
Massimo Chiari
Federica Crova
Alice Forello
Cosimo Fratticioli
Fabio Giardi
Marco Manetti
Dario Massabò
Federico Mazzei
Luca Repetto
Gianluigi Valli
Virginia Vernocchi
Franco Lucarelli
The joint use of hourly resolution sampling and analyses with accelerated ion beams such as with the particle-induced X-ray emission (PIXE) technique has allowed the measurement of hourly temporal patterns of particulate matter (PM) composition at many sites in different parts of the world. The demand within the scientific community for this type of analysis has been continuously increasing in recent years, but hourly resolution samplers suitable for PIXE analysis have been discontinued and/or suffer from some technical limitations. In this framework, a new hourly sampler, STRAS (Size- and Time-Resolved Aerosol Sampler), was developed for the collection of PM10, PM2.5 or PM1. It allows automatic sequential sampling of up to 168 hourly samples (1 week), and it is mechanically robust, compact and easily transportable. To increase PIXE sensitivity, each sample is concentrated on a small surface area on a polycarbonate membrane. Comparison between the elemental concentrations retrieved by STRAS samples and samples collected using a standard sequential sampler operated in parallel shows very good agreement; indeed, if both the samplers use the same kind of membrane, the concentrations of all detected elements are in agreement within 10 %.
- Article
(3629 KB) - Full-text XML
- BibTeX
- EndNote
Atmospheric particulate matter (PM) has been shown to represent a significant concern due to its negative effects on human health, air quality and visibility around the world (WHO, 2021; IPCC, 2023). The complexity of atmospheric aerosol properties and the variety of emission sources require advanced experimental and modeling techniques for a deeper understanding and more robust data interpretation and source apportionment.
Many studies have been devoted to PM sampling, analysis and source apportionment, using 24 h averaged data in order to have sufficient quantities of material to perform a chemical characterization that is as complete as possible and thus to obtain indications of the predominant sources of PM. However, most particulate emissions as well as atmospheric dilution processes change within a few hours. Thus, daily sampling cannot track these rapid changes. As some source emissions can strongly affect air quality, with very high loading of toxic elements during a few hours, knowledge of the timing and the intensity of specific episodes may be important for the assessment of human exposure as well as for source identification and apportionment. Furthermore, source apportionment receptor models (Belis et al., 2019) require a series of samples containing PM material from the same set of sources in different proportions: increasing the measurement time resolution typically results in samples with greater between-sample variability in the source contributions compared to samples integrated over longer time periods (Crespi et al., 2016; Crova et al., 2024).
High-time-resolution measurements (i.e., less than a few hours) require suitable techniques for both particle sampling and analysis. For time-resolved sampling, one of the most suitable solutions is to use a system that automatically switches the particle collection substrate, for example every hour, to obtain a sequence of high-time-resolution collected aerosol spots or deposits. However, this leads to a large number of “small” (in terms of mass and deposit area) collected samples to be analyzed. In this context, the particle-induced X-ray emission (PIXE) technique is an optimal analytical solution: using a properly collimated proton beam, a high number of aerosol deposits can be quickly analyzed without any sample preparation. This technique is non-destructive, multi-elemental (it allows the simultaneous detection of all the elements with Z>10) and very fast, with a high sensitivity for a small amount of matter. The signal, the X-ray yield, is indeed proportional to the areal density of the deposit: even a few micrograms can be sufficient if concentrated on a small (<1 cm2) area (Calzolai et al., 2015). Other widespread analytical techniques like ion chromatography and inductively coupled plasma mass spectrometry cannot be used to analyze this kind of sample: the only possible alternative is the use of advanced energy-dispersive X-ray fluorescence (EDXRF) spectroscopy with collimated/micro-irradiating beams or synchrotron radiation XRF, but beam availability has largely limited the development and use of this technique. It is noteworthy that among PIXE-detectable elements, there are markers of specific components such as marine aerosol (Na, Cl), mineral dust (Al, Si, Ca, Ti, Sr), sulfates (S), biomass burning (K), heavy-oil combustion (V, Ni), or traffic and industrial emission (Mn, Ni, Cu, Zn, Pb).
At the INFN LABEC ion beam laboratory (Chiari et al., 2021), a dedicated PIXE setup using a proton beam extracted into ambient pressure (external beam) has been optimized for the analysis of aerosol samples. Moreover, LABEC hosts the Elemental Mass Calibration Centre, EMC2, the reference center for measuring the mass concentration of particulate elements within the pan-European Aerosol, Clouds and Trace Gases Research Infrastructure, ACTRIS (https://www.actris-ecac.eu/, last access: 15 April 2025). The advantages of such a setup are the very short measuring time (30 or 60 s per sample) and the possibility of analyzing samples with very low mass such as those obtained with high time resolution or size-segregated samples (Lucarelli et al., 2014; Calzolai et al., 2015; Lucarelli et al., 2018).
Traditionally, high-time-resolution sampling has been carried out using streaker samplers by PIXE International Corp. (see, for example, D'Alessandro et al., 2003). Briefly, in these devices, atmospheric particles are separated onto different stages by a pre-impactor and an impactor, at a flow rate of 1 L min−1. The pre-impactor removes particles with aerodynamic diameter Dae>10 µm. The aerosol coarse fraction (2.5 µm < Dae<10 µm) is deposited on Kapton foil, which acts as the impactor stage, while the fine fraction (Dae<2.5 µm) is collected on a polycarbonate membrane. The two collecting substrata (Kapton and polycarbonate) are paired on a cartridge which rotates at a constant speed for 1 week: this produces a circular continuous deposition of particulate matter (“streak”) on both stages. PIXE analysis of these samples by a properly collimated proton beam, which scans the deposit in steps corresponding to 1 h of aerosol sampling, provides the elemental concentrations with hourly time resolution (Lucarelli et al., 2014, 2018; Calzolai et al., 2015). The “streaker + PIXE” approach has demonstrated its effectiveness in many studies (see, for example, Prati et al., 2000; D'Alessandro et al., 2003; Crespo et al., 2010; Amato et al., 2011; Dall'Osto et al., 2013; Nava et al., 2015; Lucarelli et al., 2019; Forello et al., 2019); however this sampler, designed about 40 years ago (Annegarn et al., 1988), has been discontinued.
On-line high-time-resolution instrumentation for the comprehensive analysis of aerosol composition (non-refractory components, ions, carbonaceous components, elements) is used more and more nowadays in monitoring campaigns and is available for detailed source apportionment studies. As for the elemental concentrations, which are the focus of this work, a near-real-time multi-elemental (Z>12) monitor, the Xact® 625i (by Sailbri Cooper Incorporated – SCI), has been developed. This device uses reel-to-reel filter tape sampling and energy-dispersive XRF analysis: the PM is sampled using a low-volume PM size-selective inlet (working at 1.0 m3 h−1), typically for 1–4 h, and the resulting deposit is then advanced into the measurement chamber, where it is analyzed by EDXRF for selected elements while the next sample is collected. Xact® 625i has shown good results, and its use is expanding in the aerosol research community. Nevertheless, it showed over-/underestimation of the concentration of different elements compared to reference methods (Furger et al., 2017; Tremper et al., 2018; Y. Zhu et al., 2024); the detection of light elements like Na and Mg (typical markers of fresh and aged sea salt) is not possible, and also the sensitivity to Al and Si (typical markers of soil dust) is lower compared to that of PIXE. In addition, the instrument price limits the possibility of campaigns with multiple sites in parallel, which can instead be achieved through the use of multiple cheaper samplers and subsequent PIXE analysis in one central laboratory. Finally, the X-ray source usage in the field is often subject to radioprotection restrictions by law.
In this framework, the new high-time-resolution sampler STRAS (Size- and Time-Resolved Aerosol Sampler) has been developed, as described in this paper, to replace the streaker sampler while improving the performance and technical characteristics of that previous sampler.
Extremely briefly, STRAS enables the following improvements with respect to the (discontinued) streaker: higher areal load (and so higher sensitivity for PIXE, EDXRF and optical techniques), flexibility in time resolution, the possibility of working directly with commercial US EPA (Environmental Protection Agency) inlets, and data logging for sampling parameters and for sample position.
Further, it has to be noted that STRAS allows the collection of 1 week of hourly samples without any operator intervention (or filter change); as a comparison, most of the automatic aerosol samplers allow up to about 20 samples to be loaded.
A thorough discussion of these issues is given in the following sections.
This device has been designed with the aim of obtaining a robust, reliable high-time-resolution sampler for subsequent PIXE analysis. In fact, with respect to most of the analytical techniques (such as inductively coupled plasma (ICP), ion chromatography (IC) and conventional EDXRF techniques), PIXE is much faster (allowing measurements with very good detection limits (DLs), with a measurement time down to 30 s and no need for sample pre-treatment), and thus it is the main technique applicable to a large number of samples. As mentioned, EDXRF with collimated/micro-irradiating beams or synchrotron radiation could be an option, but it is not easily available. Further comparison among the analytical techniques goes beyond the scope of this paper but may be found in the literature (e.g., Calzolai et al., 2008; Traversi et al., 2014; Yatkin et al., 2016).
The sampler has been conceived and designed in order to obtain a high-time-resolution (adjustable from 30 min to a few hours) PM10, PM2.5 and PM1 collection on small (about 1 cm2) deposit areas, with automatic sequential sampling of at least 168 samples (1 week of hourly samples) and to be mechanically robust, easy to use, compact and easily transportable.
The deposit area of each hourly sample should be small for two reasons. The first is that, as already mentioned, in PIXE spectra the signal (counts in the area of the fluorescence X-ray peaks) is proportional to the areal density of the deposit, while the continuum background is mainly due to secondary-electron bremsstrahlung radiation emitted by the collecting substratum: increasing the deposit-to-substratum thickness ratio results in a higher signal-to-noise ratio. The second reason is the necessity to limit the size of the sampler using a compact geometry: if 168 deposits are placed on a single sampling substrate compactly (like in the streaker sampler), this reduces the size of the sampler and also facilitates both sampling automation and subsequent PIXE analysis.
One of the best sampling membranes for PIXE analysis is the polycarbonate filter: it is made of very light elements which are not detectable by PIXE, it generally presents very low background values, and it is thin (about 10 µm), thus producing a low secondary-electron bremsstrahlung background in PIXE spectra (Calzolai et al., 2015). It is indeed the filtering material that has been used so far in the streaker sampler.
The deposit thickness in terms of PM areal density (µg cm−2) is given by the product of the PM concentration in air (µg cm−3); the sampling time; and the face velocity, which is equal to the flow rate divided by the deposit area. To use the sampler with easily available commercial inlets, one possible choice is a flow rate of 2.3 m3 h−1 (European standard) and another is a flow rate of 1 m3 h−1 (US standard); due to the necessity of a small deposit area, we opted for the solution with the lower flux (1 m3 h−1, i.e., 16.7 L min−1) to limit the pressure drop across the filter and the risk of filter clogging. At this flow rate, using a deposit area of 0.90 cm2, the face velocity is about 309 cm s−1, which is about 3 times the one used in the filtration stage of the streaker sampler (1 L min−1, 2×8 mm2 nozzle: v≈104 cm s−1): in these conditions, the areal density of the deposit is tripled and we obtain a gain by a factor of 3 (G=3) with respect to the streaker.
However, with this flow rate and deposit area, the pressure drop on a polycarbonate membrane can easily reach relatively high values (> 50–60 kPa), which can become difficult to sustain in continuous sampling campaigns. This difficulty has been addressed by following two approaches. First, the possibility of increasing the deposit area to 1.2 or 1.5 cm2 was implemented in STRAS, with the gain factor changing to G=2.2 or 1.8 accordingly (the same effect can also be reached keeping the 0.90 cm2 area and reducing the flow rate to 0.75 and 0.60 m3 h−1, respectively, provided that the inlets are adapted accordingly). Second, 0.8 µm pore size polycarbonate membranes (Sterlitech) were selected instead of the more frequently used 0.4 µm pore size ones (the problem of possible PM losses due to this choice is discussed in Sect. 4.1).
As a consequence of these experimental observations, the pressure drop was taken into account in the choice of the pump to be used for sampling; in the final design, the dry vacuum pump (Becker VT 4.8) was considered the best choice in terms of performance and manageability.
A cylindrical geometry, similar to that of the streaker, was chosen to minimize overall dimensions. A circular filter with a radius of 14 cm makes it possible to collect 168 samples, each one with dimensions of 3 cm (along the radial direction) by 3 to 5 mm, corresponding to a deposit area from 0.9 to 1.5 cm2. The filter is housed in a sealed cylindrical aluminum chamber (Fig. 1): the air enters from an inlet in the chamber cup, passes through the filter and exits from the suction nose; the nose is placed immediately under the filter, in contact with it to avoid flow losses (as can be verified by observing the pressure drop on the filter itself). The dimensions of the suction nose define the shape of the deposit.
The filter is coupled to a rotation system, which allows its automatic movement at every programmed time interval (1 h or other time intervals) using a controlled stepper motor (actually, the same rotation system used in the external-beam PIXE setup at LABEC). Sampling intervals longer than 1 h may be useful in remote areas, both to collect enough PM and to increase the sampler's autonomy (for example 1 month with 4 h resolution).
As regards the rest of the sampling system (inlet, cabinet, pump, airflow control system), thanks to collaboration with the Italian company Dado Lab S.r.l. (Cinisello Balsamo, Milan, Italy), it was decided to integrate STRAS into their sequential sampler Dado Lab Giano® (https://www.dadolab.com/en/products/ambient-samplers/pmx-giano-gemini, last access: 15 April 2025), replacing its sequential sampling system with the STRAS chamber. Particular attention was paid to integrating data logging for sampling parameters (airflow, pressure drop, elapsed time, etc.) and for sample position (the position of every single spot is registered and can also be reproduced in case of blackouts or stepper motor malfunctioning). While unattended, STRAS can be controlled remotely. STRAS was designed to make the use of EPA inlets possible; we used commercial single-cutoff impactors operating at 1 m (3 h)−1 with flow regulated within 2 % (available for PM10, PM2.5 and PM1).
The sampler can be easily moved: the total weight is about 36 kg, and the dimensions of the cabinet are 70 cm × 50 cm × 30 cm.
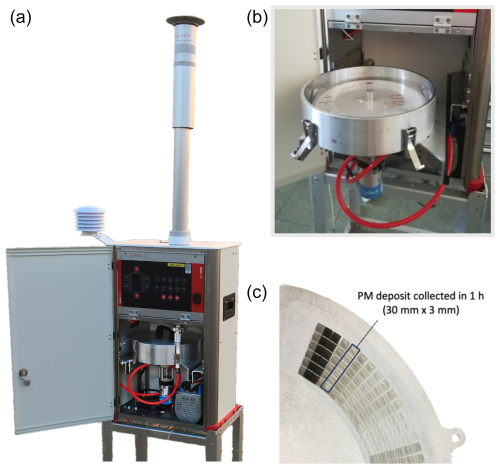
Figure 1STRAS. (a) A picture of the whole sampler: the chamber with the rotating system can be seen inside the cabinet. (b) A picture of the chamber when it is extracted (using linear guides) to be opened for inspecting or changing the filter. (c) A picture of a portion of the collecting polycarbonate membrane, where aerosol deposits can be seen.
Samples collected by STRAS can be easily analyzed by ion beam analysis (IBA) techniques, like PIXE. In Fig. 2, the analysis of a STRAS sample at the INFN LABEC external-beam PIXE setup is shown. The ion beam, typically a proton beam, can be collimated to match the aerosol spot collected in 1 h and possibly scan the spot area. No sample pre-treatment is needed. In our setup, the proton beam is extracted in air through a 500 nm Si3N4 window, and the samples are positioned at about 1 cm distance from it, perpendicularly to the beam. A collimator at the end of the beamline sets the beam spot to 1×2 mm2; the charge flown during the measurement is measured by integrating the beam current on a graphite Faraday cup positioned just behind the samples. The movement of the samples on the x–y axes (perpendicular to the beam direction) and the change of the samples (reconstruction of the time sequence) by rotation of the sample holder (i.e., the STRAS polycarbonate filter in this case) are both remotely controlled by the acquisition system. The use of high beam currents (from tens to hundreds of nanoamperes) and an optimized X-ray detection system (which includes three silicon drift detectors, one for elements Na–Ca and the other two for elements Ti–Pb) allow for the detection of all the elements with Z>10 with good sensitivity in short measuring times (Lucarelli et al., 2014, 2018; Calzolai et al., 2015; Chiari et al., 2020).
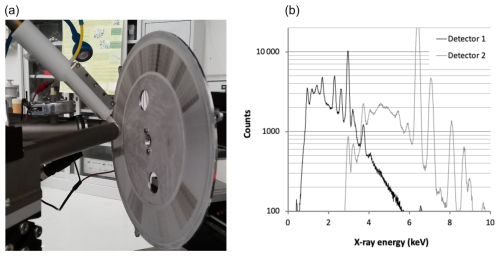
Figure 2STRAS during PIXE analysis at the INFN LABEC external-beam setup (a). PIXE spectra of a 1 h PM10 STRAS sample, simultaneously obtained using a 3 MeV proton beam in 100 s at 150 nA (b).
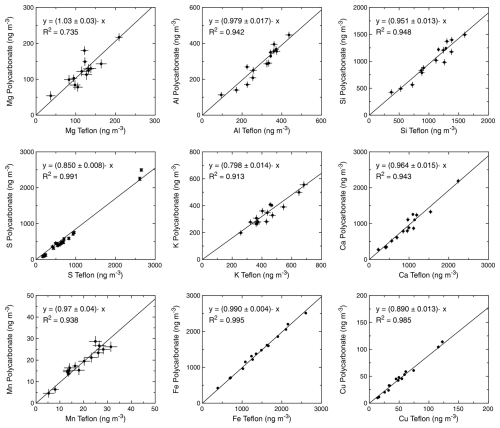
Figure 3Scatterplots of elemental concentrations of the main elements detected by PIXE in PM10, simultaneously collected by the dual-channel Gemini sampler on 0.8 µm polycarbonate and Teflon membranes. In each plot the fitted correlation line, together with the correlation coefficient, is also shown.
Several STRAS samples, both blank and loaded filters, were analyzed to evaluate the quality of the spectra and determine the detection limits (DLs). Elemental concentrations are obtained using the GUPIXWIN software (Campbell et al., 2010), using an H-factor free parameter (obtained by the analysis of a set of thin mono- and bi-elemental standards by Micromatter Technologies Inc.). The total uncertainties in elemental concentrations are determined by the sum of independent uncertainties in certified thicknesses of the standards (5 %), deposit area (5 %), airflow (2 %–5 %), X-ray peak fit and peak area counting statistics (2 % for the more abundant elements, and then this increases when the concentrations approach DLs). Measurement quality assurance checks were routinely performed (by analyzing the NIST SRM 2783). Measurements and uncertainty estimates were performed according to IBA measurements guidelines (https://www.actris-ecac.eu/pmc-elements.html, last access: 15 April 2025).
In typical measuring conditions (15 µC of integrated charge per sample, i.e., 60 s at a 250 nA proton beam current or 100 s at 150 nA), areal concentration DLs were on the order of 10–20 ng cm−2 for the low-Z elements (Na–Ca) and 1–10 ng cm−2 for medium-Z to high-Z elements (Ti–Pb). To obtain DLs for in-air concentrations (ng m−3), these values should be simply multiplied by a factor corresponding to the deposit area (0.9, 1.2 or 1.5 cm2) and divided by the sampled volume (m3).
Tests to check the reliability of the new sampler were carried out performing a field campaign where the elemental concentrations were obtained in parallel by PIXE on STRAS samples (1 h time resolution) and on samples collected by the dual-channel Gemini sampler (Dado Lab S.r.l., Italy) operated at 2 h time resolution. PM10, PM2.5 and PM1 samples were collected during wintertime in 2023 at the campus of the University of Milan, inside the Department of Physics courtyard (lat 45.47665, long 9.23082; 122 m a.s.l.), an urban background site located on the eastern side of the city. To obtain samples with sufficient deposit areal density, the Gemini sampler routinely working with 47 mm diameter membranes was equipped with specially designed inlet reducers and filter holders, which allow the use of 25 mm diameter filters, thus increasing the areal density by a factor of 3.6 (Caiazzo et al., 2021).
To avoid the comparison suffering from effects due to a different type of collecting substrate, one channel of the Gemini sampler was equipped with the same kind of membranes used for STRAS, i.e., 0.8 µm pore size polycarbonate filters. At the same time, to investigate possible PM losses due to a reduced collection efficiency of these membranes, the second channel was equipped with ring-supported thin Teflon filters, which are known to have approx. 100 % aerosol retention (Zikovà et al., 2015) and are suitable for PIXE analysis.
For each PM fraction, 20 polycarbonate samples and 20 Teflon samples were collected with 2 h resolution, and 40 STRAS 1 h samples were gathered in parallel. All the samples were analyzed at the LABEC external-beam PIXE setup as described in the previous section. STRAS hourly elemental concentrations were averaged over a 2 h time interval to be compared with the parallel samples.
4.1 Filter collection efficiency
It is well known that the pressure drop on polycarbonate filters also depends on pore size, and bigger pore diameters are generally associated with smaller pressure drops; this can be advantageous, especially when a high sampling flow rate through a small collecting area is considered, but at the same time, larger pores are also related to smaller collection efficiencies. As the STRAS flow rate and a small collecting surface require polycarbonate filters with 0.8 µm pores, the efficiency of filters was investigated.
According to the classical theory dating back to the 1960s–1970s (see, for example, Manton, 1978, 1979; Spurny et al., 1969, 1972; Chen et al., 2013), the polycarbonate filters can be parametrized through a physical model for which filters are constituted by parallel capillaries; in this way, filter parameters (pore size; filter thickness; and porosity, i.e., the ratio of open space over the total filter volume), particle parameters (size and density) and filtration conditions (e.g., the air face velocity on the filter) can be used for the calculation of particle collection efficiency. Particle collection by these filters can be described as a combination of different processes: (1) the inertial impact on the filter surface, (2) interception at the pore opening, (3) Brownian diffusion to the pore walls and (4) Brownian diffusion to the filter surface. The combination of these effects leads to a typical minimum in efficiency (Hinds, 1999), which – in the experimental conditions used in this work – has been calculated to be around 40 %–50 % for particles in the range of 50–80 nm assuming a hypothetical density equal to 2 g cm−3.
In this work, a field test was performed using parallel samples collected on polycarbonate and Teflon filters for different size fractions. The results are reported in Fig. 3 for PM10, which showed the highest aerosol mass concentrations and thus gave the most reliable values for comparison.
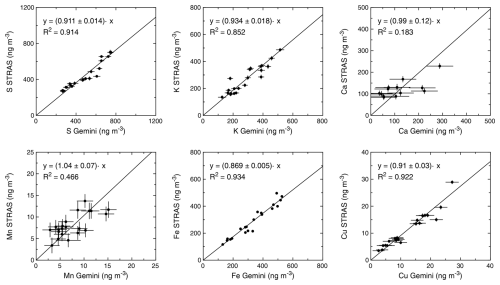
Figure 5Scatterplots of elemental concentrations of the main elements detected by PIXE in PM2.5 samples simultaneously collected by STRAS and Gemini on polycarbonate (pore size: 0.8 µm). In each plot the fitted correlation line, together with the correlation coefficient, is also shown.
In general, high correlation was found (>0.9 for all the elements except Mg), and the comparison between the two datasets shows agreement within a few percent for all the detected elements except for S and K, with deviations ranging from 15 % to 20 % – with samples on 0.8 µm polycarbonate showing lower values than those on Teflon filters. As previously mentioned, for these polycarbonate membranes, the minimum in collection efficiency is expected below 100 nm (Hinds, 1999). Thus, only elements with a size distribution strongly skewed towards the Aitken or condensation modes can be affected by significant losses. Focusing on the elements detected with PIXE (Z>10), a significant contribution to these modes (at least in urban areas) is expected for S and K only, as S is mainly present as a secondary aerosol and K may be produced by biomass burning (see, for example, Bernardoni et al., 2017b).
Similar results were obtained for PM2.5 and PM1: focusing on S and K underestimation, slightly lower slopes were found for S (PM2.5: 0.78±0.02; PM1: 0.74±0.05), while the same values within uncertainties were found for K (PM2.5: 0.84±0.03; PM1: 0.80±0.05). As for PM10, no effect was observed for the other elements.
Therefore, it should be taken into account that the polycarbonate membrane with 0.8 µm pores can likely have lower particle retention for some elements; the lower efficiency of polycarbonate membranes with respect to Teflon filters is also confirmed by other studies in the literature (Soo et al., 2016, and the cited literature therein).
4.2 STRAS field validation
The STRAS validation in the field was performed using samples collected in parallel on the same filter type to avoid, as much as possible, differences in the samples used for comparison.
In Fig. 4, STRAS versus Gemini polycarbonate scatterplots are reported for the main detected elements in PM10 samples. As can be seen from the fitting results, correlations are very good (≥0.9) for all the elements and the angular coefficients are close to 1 (equal to 1 within 10 % for all the elements, better than 5 % for most of them). For some elements, Gemini data are affected by larger uncertainties due to the lower aerosol areal concentration on the samples collected with the Gemini sampler (this also holds true for Figs. 5 and 6).
Similar results were obtained for PM2.5 and PM1 (Figs. 5 and 6), albeit with a more scattered behavior due to the lower counting statistic in fluorescence X-ray peaks (note the difference in scale ranges for PM10).
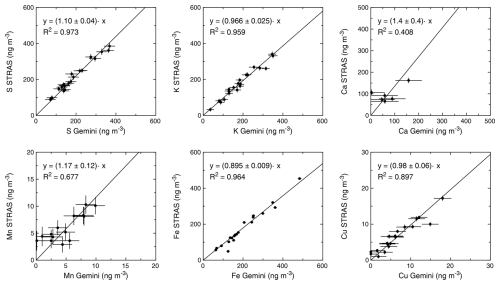
Figure 6Scatterplots of elemental concentrations of the main elements detected by PIXE in PM1 samples simultaneously collected by STRAS and Gemini on polycarbonate (pore size: 0.8 µm). In each plot the fitted correlation line, together with the correlation coefficient, is also shown.
The sampler was used to collect PM1 during the RHAPS (Redox-activity and Health-effects of Atmospheric Primary and Secondary aerosol) project (Costabile et al., 2022; Crova et al., 2024). Within this project, PM1 samples were simultaneously collected by STRAS with a 1 h time resolution and by a dual-channel Gemini sampler with a daily time resolution on ring-supported thin Teflon filters (Pall R2PJ047, i.e., the same as those used in the aforementioned tests but with a standard 47 mm diameter). This allowed a further comparison between the two samplers, although only for daily averages and with samples collected on different membrane types. Results (Fig. 7) confirmed the outcomes of the tests reported above: elemental concentrations obtained by the analysis of STRAS samples agree with those obtained by the analysis of 24 h PM samples collected on ring-supported Teflon filters, except for S and K, which in this case resulted in underestimation by about 30 %.
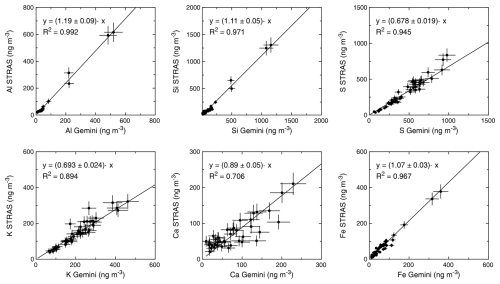
Figure 7Scatterplots of elemental concentrations of the main elements detected by PIXE in PM1 samples collected in parallel by STRAS (on 0.8 µm polycarbonate membranes) and by Gemini (on ring-supported Teflon filters). STRAS concentrations are daily-averaged to match the Gemini time resolution. In each plot the fitted correlation line, together with the correlation coefficient, is also shown.
A new high-time-resolution sampler, STRAS (Size- and Time-Resolved Aerosol Sampler), has been designed, developed and tested.
It has been conceived to collect optimal samples for subsequent PIXE analysis; the aerosol deposit is concentrated on a small surface area, thus increasing the sensitivity of the technique, and on a polycarbonate membrane, which is one of the best choices for PIXE. Compared to the streaker sampler, formerly used for retrieving 1 h resolution elemental concentrations by PIXE, the PM areal density is increased by a factor ranging from 1.8 to 3.
STRAS has the advantage of working at the standard flow rate of 1 m3 h−1 (US standard flow rate for PM10 and PM2.5 inlets) so that it can be equipped with commercial inlets for the collection of the main PM fractions (PM10, PM2.5 and PM1). It allows automatic sequential sampling of up to 168 hourly samples (1 week), and it is mechanically robust, compact and transportable.
Samples collected by STRAS can be easily analyzed by PIXE. No sample pre-treatment is needed. Using an optimized setup (high beam currents and balanced X-ray detector system), 1 min for each spot is enough to obtain good statistics: hourly samples collected in 1 week can be analyzed in 3 h of beam time.
An extensive series of tests were performed to verify the correct functioning of STRAS, through comparison with a sequential sampler used for routine PM collection (dual-channel Gemini sampler). If the same membrane (0.8 µm pore size polycarbonate) is used by both samplers, the concentrations of all detected elements agree within 10 %, thus demonstrating the reliability of STRAS.
Possible PM losses due to a reduced collection efficiency of these membranes were investigated by simultaneous sampling on ring-supported thin Teflon filters: significant effects (15 %–30 % underestimation) were observed only for S and K, which are elements typically related to smaller particles originated by secondary aerosol processes and biomass burning, respectively (and thus to size distributions which extend down to below 100 nm, where the 0.8 µm polycarbonate membranes have a minimum in collection efficiency). As a consequence, it is important to be aware that S and K concentrations can be underestimated when using these membranes. In our tests these underestimations were found to be quite stable; however, some parallel sampling, even on a daily basis, may be helpful in determining correction coefficients for other sampling sites. It should be noted that the values found in this work can be considered maximum limits of underestimation since the tests were carried out in places characterized by the strong prevalence of ultrafine aerosols, such as secondary and combustion aerosols.
Information obtained by STRAS and PIXE, i.e., the elemental composition (from Na to Pb), may be complemented by other measurements. The same STRAS samples can be analyzed by other IBA techniques (simultaneously with PIXE) and by optical techniques (before PIXE analysis to avoid possible changes in the optical properties of the samples due to sample–beam interaction). In particular, black carbon and brown carbon concentrations can be measured by multi-wavelength optical analysis (Bernardoni et al., 2017a; Massabò et al., 2015).
Furthermore, STRAS may be used “side by side” with other high-time-resolution instrumentation, like the Aerosol Chemical Speciation Monitor (ACSM) for non-refractory components (NO, SO, NH, Cl−, organic aerosol (OA)), the Aethalometer for black carbon, and the on-line Sunset field analyzer for organic carbon (OC) and elemental carbon (EC).
A two-stage version of STRAS is under development, with the fine fraction collected on a polycarbonate filter, as in the model described in this paper, and the coarse fraction collected by inertial impaction on thin polypropylene foil.
Data are available upon request to the corresponding author (calzolai@fi.infn.it).
Conceptualization of the new sampler: SN, RV, PP, VB, GC, DM, GV, FL. Mechanical and electronic implementation of the sampler: LCar, CC, MM. Aerosol sampling: VB, LCad, FC, AF, FG, GV. PIXE measurements: SN, GC, MC, FG, FL. Formal analysis: SN, FG, MC. Data analysis, validation and discussion: SN, RV, PP, VB, GC, MC, DM, FM, LR, GV, VV, FL. Project management and fundraising: FL, GC, RV. Original draft writing: SN, RV, GC. Paper review and editing: RV, PP, VB, MC, FC, CF, DM, FM, GV, FL.
The contact author has declared that none of the authors has any competing interests.
Publisher’s note: Copernicus Publications remains neutral with regard to jurisdictional claims made in the text, published maps, institutional affiliations, or any other geographical representation in this paper. While Copernicus Publications makes every effort to include appropriate place names, the final responsibility lies with the authors.
The authors acknowledge the fruitful collaboration with Dado Lab S.r.l., and in particular with Gianluca Cazzuli, Giulio Gargioni and Stefano Alberti.
The authors were supported by the National Institute for Nuclear Physics (INFN) through the experiment TRACCIA and the grant STRASPEED. This work was realized with a contribution of the Fondazione CR Firenze and the support of MIUR through the project RHAPS (grant number 2017MSN7M8). The work was supported by IR0000032 – ITINERIS, Italian Integrated Environmental Research Infrastructures System (D.D. no. CE12 130/2022 – CUP B53C22002150006) funded by the EU – NextGenerationEU PNRR, Mission 4 “Education and Research”, Component 2 “From research to business”, Investment 3.1 “Fund for the realisation of an integrated system of research and innovation infrastructures”. The work was also supported by the Italian PON “Ricerca e Innovazione 2014–2020” project PER-ACRIS-IT (PIR_01_00015). This research was supported by the Instituto Nazionale di Fisica Nucleare.
This paper was edited by Mingjin Tang and reviewed by four anonymous referees.
Amato, F., Viana, M., Richard, A., Furger, M., Prévôt, A. S. H., Nava, S., Lucarelli, F., Bukowiecki, N., Alastuey, A., Reche, C., Moreno, T., Pandolfi, M., Pey, J., and Querol, X.: Size and time-resolved roadside enrichment of atmospheric particulate pollutants, Atmos. Chem. Phys., 11, 2917–2931, https://doi.org/10.5194/acp-11-2917-2011, 2011.
Annegarn, H. J., Cahill, T. A., Sellschop, J. F. P., and Zucchiatti, A.: Time sequence particulate sampling and nuclear analysis, Physics Scripta, 37, 282–290, 1988.
Belis, C. A., Favez, O., Mircea, M., Diapouli, E., Manousakas, M.-I., Vratolis, S., Gilardoni, S., Paglione, M., Decesari, S., Mocnik, G., Mooibroek, D., Salvador, P., Takahama, S., Vecchi, R., and Paatero, P.: European guide on air pollution source apportionment with receptor models – Revised version 2019, Report EUR 29816 EN, Publications Office of the European Union, Luxembourg, 2019, ISBN 978-92-76-09001-4, https://doi.org/10.2760/439106, JRC117306, 2019.
Bernardoni, V., Valli, G., and Vecchi, R.: Set-up of a multi wavelength polar photometer for off-line absorption coefficient measurements on 1-h resolved aerosol samples, J. Aerosol Sci., 107, 84–93, https://doi.org/10.1016/j.jaerosci.2017.02.009, 2017a.
Bernardoni, V., Elser, M., Valli, G., Valentini, S., Bigi, A., Fermo, P., Piazzalunga, A., and Vecchi, R.: Size-segregated aerosol in a hot-spot pollution urban area: Chemical composition and three-way source apportionment, Environ. Pollut., 231 601–611, 2017b.
Campbell, J. L., Boyd, N. I., Grassi, N., Bonnick, P., and Maxwell, J. A.: The Guelph PIXE software package IV, Nucl. Instr. Meth. B, 268, 3356, https://doi.org/10.1016/j.nimb.2010.07.012, 2010.
Caiazzo, L., Calzolai, G., Becagli, S., Severi, M., Amore, A., Nardin, R., Chiari, M., Giardi, F., Nava, S., Lucarelli, F., Pazzi, G., Cristofanelli, P., Virkkula, A., Gambaro, A., Barbaro, E., and Traversi, R.: Carbonaceous Aerosol in Polar Areas: First Results and Improvements of the Sampling Strategies, Atmosphere, 12, 320, https://doi.org/10.3390/atmos12030320, 2021.
Calzolai, G., Chiari, M., Lucarelli, F., Mazzei, F., Nava, S., Prati, P., Valli, G., and Vecchi, R.: PIXE and XRF analysis of particulate matter samples: an inter-laboratory comparison, Nucl. Instr. Meth. B, 266, 2401–2404, 2008.
Calzolai, G., Lucarelli, F., Chiari, M., Nava, S., Giannoni, M., Carraresi, L., Prati, P., and Vecchi, R.: Improvements in PIXE analysis of hourly particulate matter samples, Nucl. Instr. Meth. B, 363, 99–104, 2015.
Chiari, M., Barone, S., Bombini, A., Calzolai G., Carraresi, L., Castelli, L., Czelusniak, C., Fedi, M. E., Gelli, N., Giambi, F., Giardi, F., Giuntini, L., Lagomarsino, S., Liccioli, L., Lucarelli, F., Manetti, M., Massi, M., Mazzinghi, A., Nava, S., Ottanelli, P., Sciortino, S., Ruberto, C., Sodi, L., Taccetti, F., and Mandò, P. A.: LABEC, the INFN ion beam laboratory of nuclear techniques for environment and cultural heritage, Eur. Phys. J. Plus, 136, 472, https://doi.org/10.1140/epjp/s13360-021-01411-1, 2021.
Costabile, F., Decesari, S., Vecchi, R., Lucarelli, F., Curci, G., Massabò, D., Rinaldi, M., Gualtieri, M., Corsini, E., Menegola, E., Canepari, S., Massimi, L., Argentini, S., Busetto, M., Di Iulio, G., Di Liberto, L., Paglione, M., Petenko, I., Russo, M., Marinoni, A., Casasanta, G., Valentini, S., Bernardoni, V., Crova, F., Valli, G., Forello, A. C., Giardi, F., Nava, S., Pazzi, G., Prati, P., Vernocchi, V., La Torretta, T., Petralia, E., Stracquadanio, M., Zanini, G., Melzi, G., Nozza, E., Iulini, M., Caruso, D., Cioffi, L., Imperato, G., Giavarini, F., Battistoni, M., Di Renzo, F., Frezzini, M. A., Perrino, C., and Facchini, M. C.: On the Redox-Activity and Health-Effects of Atmospheric Primary and Secondary Aerosol: Phenomenology, Atmosphere, 13, 704, https://doi.org/10.3390/atmos13050704, 2022.
Crespi, A, Bernardoni, V., Calzolai, G., Lucarelli, F., Nava, S., Valli, G., and Vecchi, R.: Implementing constrained multitime approach with bootstrap analysis in ME-2: An application to PM2.5 data from Florence (Italy), Sci. Total Environ., 541, 502–511, https://doi.org/10.1016/j.scitotenv.2015.08.159, 2016.
Crespo, J., Yubero, E., Nicolas, J., Lucarelli, F., Nava, S., Chiari, M., and Calzolai, G.: High-time resolution and size-segregated elemental composition in high-intensity pyrotechnic exposures, J. Hazard. Mater., 241–242, 82–91, https://doi.org/10.1016/j.jhazmat.2012.09.017, 2010.
Crova, F., Forello, A. C., Bernardoni, V., Vernocchi, V., and Vecchi, R.: Assessing the role of atmospheric dispersion vs. emission strength in the southern Po Valley (Italy) using dispersion-normalised multi-time receptor modelling, Atmos. Environ., 316, 12016, https://doi.org/10.1016/j.atmosenv.2023.120168, 2024.
D'Alessandro, A., Lucarelli, F., Mandò, P.A., Marcazzan, G., Nava, S., Prati, P., Valli, G., Vecchi, R., and Zucchiatti, A.: Hourly elemental composition and source identification of fine and coarse PM10 particulate matter in four Italian towns, J. Aerosol Sci., 34, 243–259, 2003.
Dall'Osto, M., Querol, X., Amato, F., Karanasiou, A., Lucarelli, F., Nava, S., Calzolai, G., and Chiari, M.: Hourly elemental concentrations in PM2.5 aerosols sampled simultaneously at urban background and road site during SAPUSS – diurnal variations and PMF receptor modelling, Atmos. Chem. Phys., 13, 4375–4392, https://doi.org/10.5194/acp-13-4375-2013, 2013.
Forello, A. C., Bernardoni, V., Calzolai, G., Lucarelli, F., Massabò, D., Nava, S., Pileci, R. E., Prati, P., Valentini, S., Valli, G., and Vecchi, R.: Exploiting multi-wavelength aerosol absorption coefficients in a multi-time resolution source apportionment study to retrieve source-dependent absorption parameters, Atmos. Chem. Phys., 19, 11235–11252, https://doi.org/10.5194/acp-19-11235-2019, 2019.
Furger, M., Minguillón, M. C., Yadav, V., Slowik, J. G., Hüglin, C., Fröhlich, R., Petterson, K., Baltensperger, U., and Prévôt, A. S. H.: Elemental composition of ambient aerosols measured with high temporal resolution using an online XRF spectrometer, Atmos. Meas. Tech., 10, 2061–2076, https://doi.org/10.5194/amt-10-2061-2017, 2017.
Hinds, W. C.: Aerosol technology: properties, behavior, and measurement of airborne particles, John Wiley and Sons, ISBN 978-1-119-49404-1, 1999.
IPCC: Climate Change 2023: Synthesis Report. Contribution of Working Groups I, II and III to the Sixth Assessment Report of the Intergovernmental Panel on Climate Change, edited by: Core Writing Team, Lee, H., and Romero, J., IPCC, Geneva, Switzerland, 184 pp., https://doi.org/10.59327/IPCC/AR6-9789291691647, 2023.
Lucarelli, F., Calzolai, G., Chiari, M., Giannoni, M., Mochi, D., Nava, S., and Carraresi, L.: The upgraded external-beam PIXE/PIGE set-up at LABEC for very fast measurements on aerosol samples, Nucl. Instr. Meth. B, 318, 55–59, 2014.
Lucarelli, F., Barrera, V., Becagli, S., Chiari, M., Giannoni, M., Nava, S., Traversi, R., and Calzolai, G.: Combined use of daily and hourly data sets for the source apportionment of particulate matter near a waste incinerator plant, Environ. Pollut., 247, 802–811, https://doi.org/10.1016/j.envpol.2018.11.107, 2019.
Lucarelli, L., Calzolai, G., Chiari, M., Nava, S., and Carraresi, L.: Study of atmospheric aerosols by IBA techniques: The LABEC experience, Nucl. Instr. Meth. B, 417, 121–127, 2018.
Manton, M. J.: The impaction of aerosols on a nuclepore filter, Atmos. Environ., 12, 1669–1675, 1978.
Manton, M. J.: Brownian diffusion of aerosols to the face of a nuclepore filter, Atmos. Environ., 13, 525–531, 1979.
Massabò, D., Caponi, L., Bernardoni, V., Bove, M. C., Brotto, P., Calzolai, G., Cassola, F., Chiari, M., Fedi, M. E., Fermo, P., Giannoni, M., Lucarelli, F., Nava, S., Piazzalunga, A., Valli, G., Vecchi, R., and Prati, P.: Multi-wavelength optical determination of black and brown carbon in atmospheric aerosols, Atmos. Environ., 108, 1–12, 2015.
Nava, S., Lucarelli, F., Amato, F., Becagli, S., Calzolai, G., Chiari, M., Giannoni, M., Traversi, R., and Udisti, R.: Biomass burning contributions estimated by synergistic coupling of daily and hourly aerosol composition records, Sci. Total Environ., 511, 11–20, 2015.
Prati, P., Zucchiatti, A., Lucarelli, F., and Mandò, P. A.: Source apportionment near a steel plant in Genoa by continuous aerosol sampling and PIXE analysis, Atmos. Environ., 34, 3149–3157, 2020.
Soo, J.-C., Monaghan, K., Lee, T., Kashon, M., and Harper, M.: Air sampling filtration media: Collection efficiency for respirable size-selective sampling, Aerosol Sci. Technol., 50, 76–87, 2016.
Spurny, K. R. and Lodge Jr., J. P.: Collection Efficiency Tables for Membrane Filters Used in the Sam-pling and Analysis of Aerosols and Hydrosols, NCAR Technical Note NCAR/TN-77+STR, University Corporation for Atmospheric Research, https://doi.org/10.5065/D6F769JJ, 1972.
Spurny, K. R., Lodge, J. P., Frank, E. R., and Sheesley, D. C.: Aerosol Filtration byMeans of Nuclepore Filters: Structural and Filtration Properties, Environ. Sci. Technol., 3, 453–464, 1969.
Traversi, R., Becagli, S., Calzolai, G., Chiari, M., Giannoni, M., Lucarelli, F., Nava, S., Rugi, F., Severi, M., and Udisti, R.: A comparison between PIXE and ICP-AES measurements of metals in aerosol particulate collected in urban and marine sites in Italy, Nucl. Instr. Meth. B, 318, 130–134, 2014.
Tremper, A. H., Font, A., Priestman, M., Hamad, S. H., Chung, T.-C., Pribadi, A., Brown, R. J. C., Goddard, S. L., Grassineau, N., Petterson, K., Kelly, F. J., and Green, D. C.: Field and laboratory evaluation of a high time resolution x-ray fluorescence instrument for determining the elemental composition of ambient aerosols, Atmos. Meas. Tech., 11, 3541–3557, https://doi.org/10.5194/amt-11-3541-2018, 2018.
WHO global air quality guidelines: Particulate matter (PM2.5 and PM10), ozone, nitrogen dioxide, sulfur dioxide and carbon monoxide, Geneva: World Health Organization, ISBN 9789240034228, https://www.who.int/publications/i/item/9789240034228 (last access: 5 May 2025), 2021.
Yatkin, S., Belis, C. A., Gerboles, M., Calzolai, G., Lucarelli, F., Cavalli, F., and Trzepla, K.: An interlaboratory comparison study on the measurement of elements in PM10, Atmos. Environ., 125, 61–68, 2016.
Zhu, Y., Liu, C., Huo, J., Li, H., Chen, J., Duan, Y., and Huang, K.: A novel calibration method for continuous airborne metal measurements: Implications for aerosol source apportionment, Sci. Total Environ., 908, 168274, https://doi.org/10.1016/j.scitotenv.2023.168274, 2024.
Zíková, N., Ondráček, J., and Ždímal, V.: Size-Resolved Penetration Through High-Efficiency Filter Media Typically Used for Aerosol Sampling, Aerosol Sci. Technol., 49, 239–249, https://doi.org/10.1080/02786826.2015.1020997, 2015.