the Creative Commons Attribution 4.0 License.
the Creative Commons Attribution 4.0 License.
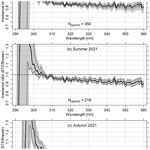
Comparison of global UV spectral irradiance measurements between a BTS CCD-array and a Brewer spectroradiometer
Carmen González
José M. Vilaplana
José A. Bogeat
Antonio Serrano
Spectral measurements of UV irradiance are of great importance for protecting human health as well as for supporting scientific research. To perform these measurements, double monochromator scanning spectroradiometers are the preferred devices thanks to their linearity and stray-light reduction. However, because of their high cost and demanding maintenance, CCD-array-based spectroradiometers are increasingly used for monitoring UV irradiance. Nevertheless, CCD-array spectroradiometers have specific limitations, such as a high detection threshold or stray-light contamination. To overcome these challenges, several manufacturers are striving to develop improved instrumentation. In particular, Gigahertz-Optik GmbH has developed the stray-light-reduced BTS2048-UV-S spectroradiometer series (hereafter “BTS”). In this study, the long-term performance of the BTS and its seasonal behavior, regarding global UV irradiance, was assessed. To carry out the analysis, BTS irradiance measurements were compared against measurements from the Brewer MK-III #150 scanning spectrophotometer during three campaigns. A total of 711 simultaneous spectra, measured under cloud-free conditions and covering a wide range of solar zenith angles (SZAs; from 14 to 70∘) and UV indexes (from 2.4 to 10.6), were used for the comparison. During the three measurement campaigns, the global UV spectral ratio BTS / Brewer was almost constant (at around 0.93) in the 305–360 nm region for SZAs below 70∘. Thus, the BTS calibration was stable during the whole period of study (∼ 1.5 years). Likewise, it showed no significant seasonal or SZA dependence in this wavelength region. Regarding the UV index, a good correlation between the BTS and the Brewer #150 was found, i.e., the dynamic range of the BTS is comparable to that of the Brewer #150. These results confirm the quality of the long-term performance of the BTS array spectroradiometer in measuring global UV irradiance.
- Article
(4068 KB) - Full-text XML
- BibTeX
- EndNote
Prolonged exposure to solar UV radiation has adverse effects on the eye, immune system, and skin of humans (Cullen et al., 1984; Armstrong and Kricker, 1993; Garssen et al., 1996) and animals alike (Kripke, 1974; Doughty and Cullen, 1990; Eller et al., 1994) given that UV photons may damage DNA, proteins and lipids (Beukers and Berends, 1960; Häder and Brodhun, 1991; Ogura et al., 1991). Moreover, this radiation can also be harmful to materials (Lawrence and Weir, 1973; Hon and Chang, 1984; Capjack et al., 1994; Andrady et al., 2019) and numerous species such as forests (Sullivan and Teramura, 1988; Musil and Wand, 1993), phytoplankton (Smith et al., 1980; Döhler and Biermann, 1987; Ekelund, 1990) and crops (Caldwell, 1968; Teramura, 1980; Krupa and Kickert, 1989). Spectral measurements are needed to determine the risks associated with UV radiation since its biological effects depend greatly on the wavelength. Furthermore, these measurements are also necessary for monitoring the short- and long-term trends of solar UV radiation (Zerefos et al., 2012; Fountoulakis et al., 2016), for testing radiative transfer models (Mayer et al., 1997) and for validating satellite products (Eck et al., 1995; Kazantzidis et al., 2006; Arola et al., 2009; Antón et al., 2010). In addition, they are also used to study the effect of ozone, clouds and atmospheric aerosols on the irradiance that reaches the earth's surface (Bernhard et al., 2007; Seckmeyer et al., 2008).
Double monochromator scanning spectroradiometers are the preferred devices for measuring UV spectral radiation due to their stray-light reduction and linearity. However, their high economic cost, slow scanning, difficulty to transport and demanding maintenance limit their large-scale deployment. In this framework, the new cost-effective spectroradiometers, based on CCD sensors, appear as an interesting alternative because of their fast scanning and compact design. However, as CCD-array spectroradiometers are single monochromators, they are significantly affected by stray light. Consequently, they require either mathematical (Zong et al., 2006; Nevas et al., 2014) or experiment-based (Jäkel et al., 2007; Shaw and Goodman, 2008) corrections to provide accurate solar UV measurements. Furthermore, the array detectors have low sensitivity (Edwards and Monks, 2003; Jäkel et al., 2007), resulting in a higher detection threshold. To improve their performance, new guidelines and techniques have been developed within several research projects such as the EMRP project ENVO3 “Traceability for surface spectral solar ultraviolet radiation” (Blumthaler et al., 2013; Nevas et al., 2014; Egli et al., 2016) and the EMRP ENV59 “Traceability for atmospheric total column ozone” (Gröbner et al., 2017; Sildoja et al., 2018; Vaskuri et al., 2018).
To overcome the aforementioned challenges, several manufacturers are devoting considerable efforts to the development of improved instrumentation. In particular, Gigahertz-Optik GmbH has developed the BTS2048-UV-S series CCD-array spectroradiometers (hereafter “BTS”). Thanks to a hardware-based stray-light correction and a BiTec Sensor, it measures spectral UV irradiance with good linearity and stray-light reduction (Zuber et al., 2018a).
Several studies have been carried out to assess the quality of the BTS series. Its performance, regarding total ozone column values, is comparable to that provided by Dobson and Brewer instruments (Zuber et al., 2018a, 2021). The UV index values derived from the BTS spectra were within ±1 % for solar zenith angles (SZAs) smaller than 70∘ in reference to a scanning DTMc300 double monochromator (Zuber et al., 2018b). Additionally, the BTS can measure both direct and global spectral irradiance with a similar quality to that obtained by the double monochromator QASUME (Quality Assurance of Spectral Solar UV Measurements in Europe) (Bais et al., 2003) and the scanning DTMc300 double monochromator (Zuber et al., 2018a, b).
Nonetheless, in these previous works, only the short-term performance of the BTS concerning global UV spectral irradiance was studied. Hence, the range of SZA and the intensity covered were narrow, limiting the complete evaluation of the stability and dynamic range of the BTS spectroradiometer. Furthermore, since the BTS has been characterized during short-term comparison campaigns, its seasonal behavior has yet to be evaluated.
Thus, the original contribution of this paper is the study of the long-term performance of the BTS regarding global UV spectral irradiance. The study also analyzes the diurnal and seasonal dependence of the sensitivity as well as the performance of the BTS measuring the UV index. The results obtained contribute greatly toward quantifying the quality of the BTS measurements.
The paper is organized as follows. The characteristics of the spectrometers Brewer #150 and BTS used in this work are described in Sect. 2. Next, Sect. 3 presents the methodology applied to compare the spectral irradiance of both instruments. In Sect. 4 the spectral irradiance and UV index ratio (BTS / Brewer) are analyzed. Finally, Sect. 5 summarizes the main conclusions.
The spectrometers Brewer #150 and BTS2048-UV-S-WP used in this study are installed at the El Arenosillo Atmospheric Sounding Station, located in Mazagón, Huelva (Spain). The station belongs to the Earth Observation, Remote Sensing and Atmosphere Department of the National Institute of Aerospace Technology (INTA). Every 2 years, it hosts the Regional Brewer Calibration Center – Europe (RBCC-E) intercomparison campaigns, where Brewers are calibrated for total ozone column (TOC) and global UV irradiance.
2.1 Brewer #150
The Brewer MK-III #150 is a double monochromator spectrophotometer that measures global UV spectral irradiance between 290 and 363 nm with a step of 0.5 nm. It has a full width half maximum (FWHM) of 0.6 nm and a wavelength accuracy of 0.05 nm. In this configuration, a complete scan takes approximately 4.5 min. Instead of the traditional design (a standard flat diffuser), the Brewer #150 features a CMS-Schreder entrance optic (Model UV-J1015) which improves the angular response, reproducibility and accuracy of global irradiance measurements. This diffuser and the optics were aligned and finely adjusted in October 2019. The resulting angular response was accurately measured in the laboratory, obtaining an integrated cosine error f2 of 1.4 %.
The spectroradiometer is calibrated every 2 years for solar UV irradiance against the European traveling reference QASUME B5503 (Hülsen et al., 2016), following the methodology set by the Physikalisch-Meteorologisches Observatorium Davos, World Radiation Center (https://projects.pmodwrc.ch/qasume/qasume_audit/reports/, last access: 27 June 2022). Additionally, it is periodically calibrated with several quartz-halogen standard lamps (1000 W DXW type). Thanks to these calibrations, the quality and accuracy of the UV spectral irradiance measured by the Brewer #150 are guaranteed.
2.2 BTS2048-UV-S-WP
The BTS2048-UV-S-WP is a CCD-array spectroradiometer manufactured by Gigahertz-Optik GmbH. One of its most important features is the BiTec Sensor (BTS), which combines the properties of an integral detector with those of a spectral detector, resulting in high-quality measurements.
The spectral detector is based on a cooled back-thinned CCD detector with 2048 pixels and an electronic shutter (Zuber et al., 2018a, b). It exhibits an FWHM of 0.8 nm, a pixel resolution of 0.13 nm per pixel and a spectral range of 190–430 nm. The CCD has an integration time that ranges from 2 µs to 60 s. On the other hand, the integral detector consists of a silicon carbide (SiC) photodiode with measurement time ranging from 0.1 ms to 6 s. Since the spectroradiometer is designed for outdoor measurements, it is contained in weather-proof housing which removes humidity and controls temperature to 38 ∘C. Regarding the input optics, the BTS2048-UV-S-WP features a cosine-corrected diffuser window to improve its angular response, sensitivity and calibration stability.
To overcome the issues faced with most array spectroradiometers due to the internal stray light, the BTS spectroradiometer is equipped with several optical filters mounted on a remote-controlled filter wheel (described in detail by Zuber et al., 2018a), ruling out the need for mathematical stray-light correction methods.
To validate the long-term performance of the BTS, three campaigns measuring global UV spectral irradiance were carried out at the El Arenosillo Atmospheric Sounding Station. The first campaign was conducted from 26 May to 16 June 2020 (spring 2020), the second one from 5 to 15 July 2021 (summer 2021) and the third one from 10 to 25 November 2021 (autumn 2021).
During the three campaigns different atmospheric conditions were observed, with cloud-free, partly overcast and totally overcast skies being covered. However, only cloud-free conditions were considered in order to reliably compare the almost instantaneous spectrum measured by the BTS with the low-scanned spectrum of the Brewer. Furthermore, the comparison was also limited to SZAs lower than 70∘ to avoid possible issues related to the cosine error, whose contribution can be significant at large SZAs. Regarding the ozone, throughout the spring 2020 campaign it varied from 290 to 333 DU, during the summer 2021 campaign it ranged from 284 to 324 DU and in the autumn of 2021 it fluctuated between 280 and 325 DU. Regarding SZA coverage, the minimum SZA reached was 13.8, 14.5 and 54.4∘ during the spring 2020, summer 2021 and autumn 2021 campaigns, respectively. Finally, the UV index ranged from 5.4 to 10.6 in the spring 2020 campaign, from 8.5 to 10.5 through summer 2021 and from 2.4 to 3.3 during the autumn 2021 campaign. This information is summarized in Table 1.
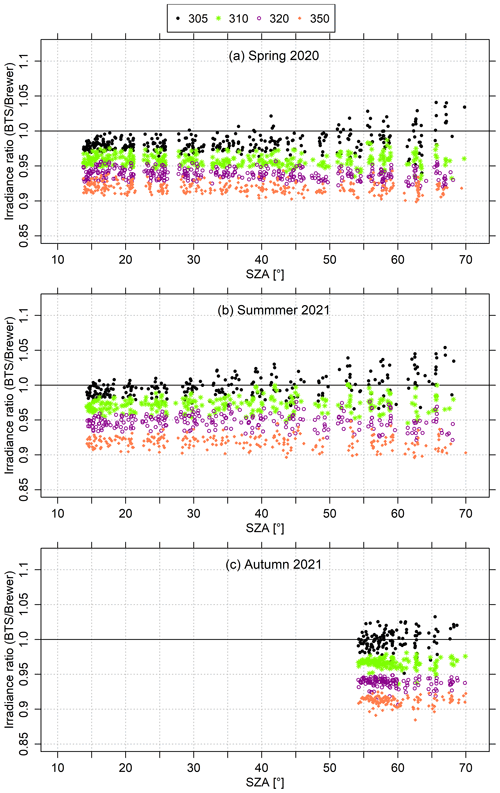
Figure 3The ratios of global UV spectral irradiance at selected wavelengths between the BTS and the Brewer #150. The measurements were obtained from cloud-free conditions and SZAs below 70∘ during (a) spring 2020, (b) summer 2021 and (c) autumn 2021. Each data point is calculated from the average over a ±2.5 nm wavelength band.
Table 2Summary statistics of the three measurement campaigns with the BTS spectroradiometer relative to the double Brewer spectrometer. The variability is defined as the difference between the 5th and the 95th percentile of all scans.
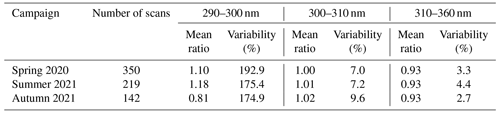
To compare the data registered by both instruments, the measured spectra had to be previously synchronized in time. As mentioned earlier, the BTS is able to record a full scan within seconds (one timestamp for one complete spectrum) whereas the Brewer takes about 4.5 min (a timestamp for each wavelength scanned). To synchronize the scans, only the BTS spectra within ±1 min of the Brewer's central wavelength (326.5 nm) timestamp were considered. However, to further improve the results, different synchronization criteria were applied to study the UV index and angular dependence of the BTS. In this way, to obtain the UV index, only the BTS spectra within ±1 min of the Brewer's 307 nm timestamp were considered. This wavelength was selected since the erythemally weighted irradiance peaks between 306 and 308 nm, depending on the SZA and total ozone. To analyze the angular dependence, the spectral ratio of BTS / Brewer was calculated in four different wavelength bands. For each band, the ratio was obtained using BTS spectra within ±1 min of the central wavelength (305, 310, 320 and 350 nm) of each band. On the other hand, to limit the number of data obtained during the campaigns, the BTS and Brewer were scheduled to measure every 2 and 15 min, respectively. Putting the former criteria into practice resulted in 350, 219 and 142 simultaneous UV spectra for the spring 2020, summer 2021 and autumn 2021 campaigns, respectively.
Finally, since both instruments have different optical bandwidths (FWHM), the measured spectra were first deconvolved with their individual slit function, and then convolved with a 1 nm triangular bandpass using the SHICRivm software package V. 3.075. This methodology also corrects the wavelength shift of the two instruments with an accuracy of 0.02 nm (Slaper et al., 1995).
4.1 Spectral analysis
To assess the long-term spectral performance of the BTS, the spectral ratios between the synchronized irradiance measurements of the BTS and the Brewer #150 reference are obtained for each measurement campaign. The data cover all SZAs lower than 70∘, and only spectra measured under cloud-free conditions are considered. The average spectral ratio between the BTS and the Brewer #150 for the wavelength range 290–360 nm is shown in Fig. 1 for the spring 2020, summer 2021 and autumn 2021 comparison campaigns.
It can be seen from Fig. 1 that the spectral ratio displays a similar behavior during the three comparison campaigns. The BTS shows a steady underestimation of global irradiance of about −7 % between 310 and 360 nm. For the other wavelength regions, the spectral ratio decreases between 300 and 310 nm. At shorter wavelengths, below 300 nm, the ratio increases rapidly and deviates by more than 20 %. This increase in the ratio could be partly due to stray light and cosine response. Although both instruments are equipped with improved diffusers and stray-light reduction, their contribution cannot be totally neglected. The wavelength threshold of reliable recording, 300 nm, is similar to other stray-light-corrected CCD-array spectroradiometers (Ylianttila et al., 2005; Ansko et al., 2008; Kouremeti et al., 2008; Egli et al., 2016). Overall, the agreement between the two instruments is satisfactory between 305 and 360 nm, as the spectral ratio varies within 5 %.
For each campaign, the variability, defined as the difference between the 5th and 95th percentile, and the mean of the spectral ratio are given in Table 2, separately for the three observed wavelength regions in Fig. 1. Table 2 confirms the previous statement: the two instruments agree within 5 % for the region between 305 and 360 nm. On the other hand, the 290–300 nm region has the largest variability. This was expected since in this wavelength range, the spectral ratio varies significantly.
Figure 1 shows that the average ratio is significantly lower for the autumn 2021 campaign exclusively in the region 290–300 nm. This behavior could be likely related to several factors, such as stray light, differences in the detection threshold between Brewer and BTS and the BTS noise reduction filter. These factors have a larger effect for low signals, which are more frequent during autumn due to the lower range of solar elevation as compared with the other two campaigns.
To check the BTS stability, the average ratios between the BTS and the Brewer #150 for the three comparison campaigns are represented together in Fig. 2. The curvature observed in Fig. 2 could be produced by several factors such as calibration sources, cosine error, stray light or the ratio's sensitivity to small variations. Except for the aforementioned differences observed at short wavelengths (below 297 nm), the ratios during the three campaigns are virtually identical. Therefore, the BTS calibration was stable during the whole study period (more than 1 year), despite the fact that no calibration checks were performed during this time. Furthermore, the BTS shows no seasonal dependence.
The spectral ratios in Figs. 1 and 2 are averages of all spectra with sufficient synchronization in time, and as a result they may be biased by systematic diurnal variations. To further describe the performance of the BTS, the ratios between the BTS and Brewer #150 are shown in Fig. 3 for different wavelength bands with respect to SZA. The ratios are averaged in ±2.5 nm wavelength bands at 305, 310, 320 and 350 nm. Wavelengths below 300 nm were not considered since at this wavelength region the ratio increases sharply (see Figs. 1 and 2).
Figure 3 shows that the spectral ratio at 305 nm has a slight dependence on SZA, increasing with growing SZA. The signal-to-noise ratio is especially low for short wavelengths according to the spectral distribution of the solar spectrum. This decrease is particularly strong for high SZAs since the radiation is attenuated as it traverses a larger path through the atmosphere. At longer wavelengths, over 310 nm, the ratios are very stable, to within less than 10 % and close to unity. In fact, the BTS shows no diurnal variation in any of the measurement campaigns. As expected, the spectral ratio slightly decreases as wavelength increases, displaying the same behavior shown in Fig. 1. These differences may be partly due to remaining stray light, cosine response and the different calibration sources for the two instruments. Furthermore, the ratio is nearly identical in all three campaigns, confirming that the BTS shows no seasonal behavior.
4.2 UV index
To evaluate the dynamic range of the BTS, an integrated quantity such as the UV index is analyzed for SZAs less than 70∘. Figure 4 represents, as a function of SZA, the daily variation of the ratios between the UV index measured by the BTS and the Brewer #150 for the three measurement campaigns. The figure reveals that the ratio is very stable and close to unity. Overall, the BTS slightly underestimates the UV index, with an average bias of less than 2 % for SZAs below 70∘. However, one should note that this bias is higher for the autumn 2021 campaign, less than 3 %, arising from the decrease in the spectral ratio between 290 and 300 nm.
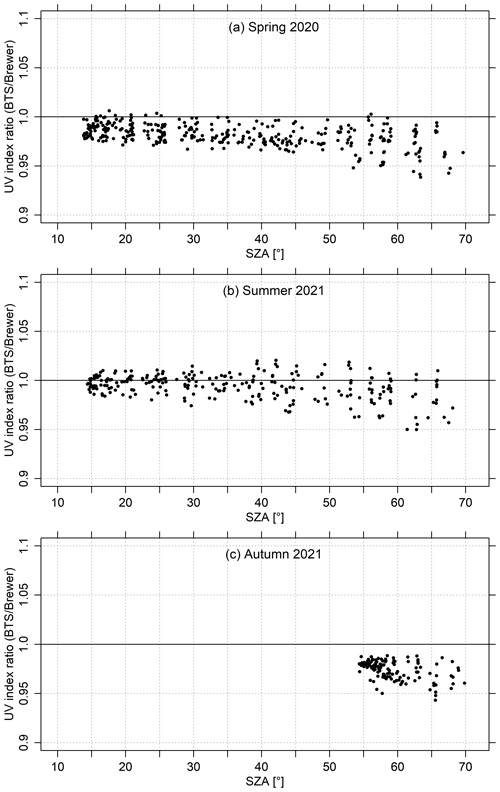
Figure 4The ratio of UV indices between the BTS and the Brewer #150 as a function of solar zenith angle. The measurements were obtained from cloud-free conditions and SZAs lower than 70∘ during (a) spring 2020, (b) summer 2021 and (c) autumn 2021.
Finally, the UV index values derived from the BTS are compared with the values obtained from the Brewer #150 (see Fig. 5). A clear linear relationship between the two instruments is found for the UV index, with a coefficient of determination close to unity. Furthermore, the slope is close to unity, (0.9945 ± 0.0013), and the intercept is close to 0 (−0.038 ± 0.008). This confirms that the BTS underestimates marginally the UV index and that its dynamic range is comparable to that of the Brewer #150.
The BTS2048-UV-S-WP long-term performance, regarding global UV spectral irradiance, was studied via three measurement campaigns and compared with a reference such as the double spectroradiometer Brewer #150.
Evaluations of the spectral ratios between the BTS and the Brewer #150 showed that the agreement between the two instruments is satisfactory between 305 and 360 nm, as the spectral ratio is constant, at around 0.94, and agrees within 5 %. At shorter wavelengths, below 300 nm, the BTS is unable to detect UV radiation with the same quality as the Brewer #150 probably due to remaining stray light and cosine response of the two instruments. This highlights the limitations of the BTS array spectroradiometer to accurately measure the entire UV-B (290–315 nm) range. Furthermore, the comparison of the three average ratios of BTS / Brewer obtained throughout each campaign reveals that the BTS has a stable calibration as well as no seasonal behavior. However, calibration checks or recalibrations are advised to ensure the correct functioning of the instrument.
On the other hand, the analysis of the variation in the spectral ratios illustrates a slight dependence on SZA for wavelengths shorter than 305 nm. At longer wavelengths, no significant dependence on SZA is found. The ratios were stable, to within less than 10 % and close to unity. Thus, solar UV measurements from the BTS and Brewer #150 spectroradiometers are very consistent.
Regarding the UV index, the BTS slightly underestimates this integrated quantity, with an average bias of less than 3 % for all SZAs below 70∘. Therefore, the BTS is able to provide reliable measurements of the UV index, an important parameter for informing the public about the impact that UV has on human health. Moreover, the BTS bias could be further improved with regular calibrations. The comparison between the UV index values measured by the BTS and the Brewer #150 showed that the dynamic range of the BTS is similar to that of the Brewer #150.
These evaluations confirmed that the BTS long-term performance of global UV spectral measurements, with its default calibration, has a quality comparable to that provided by a double monochromator Brewer spectrophotometer in the 305–360 nm region. Additionally, this study highlights the necessity of intercomparison campaigns to assess the performance of array spectroradiometers. Furthermore, it also shows the importance of repeated site comparisons to evaluate the quality of long-term UV monitoring, calibration stability, seasonal dependence and dynamic range of the spectroradiometer under study. Once their quality is assessed, array spectroradiometers could contribute to the expansion of worldwide solar UV monitoring networks.
The data and code used in this study will be provided after personal communication with the authors of the presented paper.
CG prepared the manuscript with contributions from all co-authors, developed the code and analyzed the data as part of her doctoral thesis. JAB installed the BTS and assisted in its configuration and data acquisition. JMV and AS participated in the conceptualization and provided valuable feedback on the data analysis as well as on the writing of the paper.
The contact author has declared that none of the authors has any competing interests.
Publisher’s note: Copernicus Publications remains neutral with regard to jurisdictional claims in published maps and institutional affiliations.
This work is part of the R+D+i grants (grant nos. RTI 2018-097332-B-C21 and RTI 2018-097332-B-C22) funded by MCIN/AEI/10.13039/501100011033/ and “ERDF A Way of Doing Europe”, as well as part of the projects GR18097 and IB18092 funded by Junta de Extremadura and “ERDF A Way of Doing Europe”.
This paper was edited by Udo Friess and reviewed by two anonymous referees.
Andrady, A. L., Pandey, K. K., and Heikkilä, A. M.: Interactive effects of solar UV radiation and climate change on material damage, Photoch. Photobio. Sci., 18, 804–825, https://doi.org/10.1039/C8PP90065E, 2019.
Ansko, I., Eerme, K., Lätt, S., Noorma, M., and Veismann, U.: Study of suitability of AvaSpec array spectrometer for solar UV field measurements, Atmos. Chem. Phys., 8, 3247–3253, https://doi.org/10.5194/acp-8-3247-2008, 2008.
Antón, M., Cachorro, V. E., Vilaplana, J. M., Toledano, C., Krotkov, N. A., Arola, A., Serrano, A., and de la Morena, B.: Comparison of UV irradiances from Aura/Ozone Monitoring Instrument (OMI) with Brewer measurements at El Arenosillo (Spain) – Part 1: Analysis of parameter influence, Atmos. Chem. Phys., 10, 5979–5989, https://doi.org/10.5194/acp-10-5979-2010, 2010.
Armstrong, B. K. and Kricker, A.: How much melanoma is caused by sun exposure?, Melanoma Res., 3, 395–401, https://doi.org/10.1097/00008390-199311000-00002, 1993.
Arola, A., Kazadzis, S., Lindfors, A., Krotkov, N., Kujanpää, J., Tamminen, J., Bais, A., di Sarra, A., Villaplana, J. M., Brogniez, C., Siani, A. M., Janouch, M., Weihs, P., Webb, A., Koskela, T., Kouremeti, N., Meloni, D., Buchard, V., Auriol, F., Ialongo, I., Staneck, M., Simic, S., Smedley, A., and Kinne, S.: A new approach to correct for absorbing aerosols in OMI UV, Geophys. Res. Lett., 36, L22805, https://doi.org/10.1029/2009GL041137, 2009.
Bais, A., Blumthaler, M., Gröbner, J., Seckmeyer, G., Webb, A. R., Görts, P., Koskela, T., Rembges, D., Kazadzis, S., Schreder, J., Cotton, P., Kelly, P., Kouremeti, N., Rikkonen, K., Studemund, H., Tax, R., and Wuttke, S.: Quality assurance of spectral ultraviolet measurements in Europe through the development of a transportable unit (QASUME), in: Ultraviolet Ground- and Space-Based Measurements, Models, and Effects II, edited by: Gao, W., Herman, J. R., Shi, G., Shibasoki, K., and Slusser, J. R., SPIE, 4896, 232–238, https://doi.org/10.1117/12.468641, 2003.
Bernhard, G., Booth, C. R., Ehramjian, J. C., Stone, R., and Dutton, E. G.: Ultraviolet and visible radiation at Barrow, Alaska: Climatology and influencing factors on the basis of version 2 National Science Foundation network data, J. Geophys. Res.-Atmos., 112, D09101, https://doi.org/10.1029/2006JD007865, 2007.
Beukers, R. and Berends, W.: Isolation and identification of the irradiation product of thymine, Biochim. Biophys. Acta, 41, 550–551, https://doi.org/10.1016/0006-3002(60)90063-9, 1960.
Blumthaler, M., Gröbner, J., Egli, L., and Nevas, S.: A guide to measuring solar UV spectra using array spectroradiometers, AIP Conf. Proc., 1531, 805–808, https://doi.org/10.1063/1.4804892, 2013.
Caldwell, M. M.: Solar Ultraviolet Radiation as an Ecological Factor for Alpine Plants, Ecol. Monogr., 38, 243–268, https://doi.org/10.2307/1942430, 1968.
Capjack, L., Kerr, N., Davis, S., Fedosejevs, R., Hatch, K. L., and Markee, N. L.: Protection of Humans from Ultraviolet Radiation through the Use of Textiles: A Review, Fam. Consum. Sci. Res. J., 23, 198–218, https://doi.org/10.1177/1077727X94232007, 1994.
Cullen, A. P., Chou, B. R., Hall, M. G., and Jany, S. E.: Ultraviolet-B Damages Corneal Endothelium, Am. J. Optom. Phys. Opt., 61, 473–478, https://doi.org/10.1097/00006324-198407000-00009, 1984.
Döhler, G. and Biermann, I.: Effect of u.v.-B irradiance on the response of 15 N-nitrate uptake of Lauderia annulate and Synedra planctonica, J. Plankton Res., 9, 881–890, https://doi.org/10.1093/plankt/9.5.881, 1987.
Doughty, M. J. and Cullen, A. P.: LONG-TERM EFFECTS OF A SINGLE DOSE OF ULTRAVIOLET-B ON ALBINO RABBIT CORNEA–II. DETURGESCENCE and FLUID PUMP ASSESSED in vitro, Photochem. Photobiol., 51, 439–449, https://doi.org/10.1111/j.1751-1097.1990.tb01735.x, 1990.
Eck, T. F., Bhartia, P. K., and Kerr, J. B.: Satellite estimation of spectral UVB irradiance using TOMS derived total ozone and UV reflectivity, Geophys. Res. Lett., 22, 611–614, https://doi.org/10.1029/95GL00111, 1995.
Edwards, G. D. and Monks, P. S.: Performance of a single-monochromator diode array spectroradiometer for the determination of actinic flux and atmospheric photolysis frequencies, J. Geophys. Res.-Atmos., 108, 8546, https://doi.org/10.1029/2002jd002844, 2003.
Egli, L., Gröbner, J., Hülsen, G., Bachmann, L., Blumthaler, M., Dubard, J., Khazova, M., Kift, R., Hoogendijk, K., Serrano, A., Smedley, A., and Vilaplana, J.-M.: Quality assessment of solar UV irradiance measured with array spectroradiometers, Atmos. Meas. Tech., 9, 1553–1567, https://doi.org/10.5194/amt-9-1553-2016, 2016.
Ekelund, N. G. A.: Effects of UV-B radiation on growth and motility of four phytoplankton species, Physiol. Plantarum, 78, 590–594, https://doi.org/10.1111/j.1399-3054.1990.tb05246.x, 1990.
Eller, M. S., Yaar, M., and Gilchrest, B. A.: DNA damage and melanogenesis, Nature, 372, 413–414, https://doi.org/10.1038/372413a0, 1994.
Fountoulakis, I., Bais, A. F., Fragkos, K., Meleti, C., Tourpali, K., and Zempila, M. M.: Short- and long-term variability of spectral solar UV irradiance at Thessaloniki, Greece: effects of changes in aerosols, total ozone and clouds, Atmos. Chem. Phys., 16, 2493–2505, https://doi.org/10.5194/acp-16-2493-2016, 2016.
Garssen, J., Goettsch, W., de Gruijl, F., and van Loveren, H.: Risk Assessment of UVB Effects on Resistance to Infectious Diseases, Photochem. Photobiol., 64, 269–274, https://doi.org/10.1111/j.1751-1097.1996.tb02457.x, 1996.
Gröbner, J., Kröger, I., Egli, L., Hülsen, G., Riechelmann, S., and Sperfeld, P.: The high-resolution extraterrestrial solar spectrum (QASUMEFTS) determined from ground-based solar irradiance measurements, Atmos. Meas. Tech., 10, 3375–3383, https://doi.org/10.5194/amt-10-3375-2017, 2017.
Häder, D. P. and Brodhun, B.: Effects of Ultraviolet Radiation on the Photoreceptor Proteins and Pigments in the Paraflagellar Body of the Flagellate, Euglena gracilis, J. Plant Physiol., 137, 641–646, https://doi.org/10.1016/S0176-1617(11)81215-0, 1991.
Hon, D. N.-S., and Chang, S.-T.: Surface degradation of wood by ultraviolet light, J. Polym. Sci. Pol. Chem., 22, 2227–2241, https://doi.org/10.1002/pol.1984.170220923, 1984.
Hülsen, G., Gröbner, J., Nevas, S., Sperfeld, P., Egli, L., Porrovecchio, G., and Smid, M.: Traceability of solar UV measurements using the Qasume reference spectroradiometer, Appl. Opt., 55, 7265, https://doi.org/10.1364/ao.55.007265, 2016.
Jäkel, E., Wendisch, M., Blumthaler, M., Schmitt, R., and Webb, A. R.: A CCD spectroradiometer for ultraviolet actinic radiation measurements, J. Atmos. Ocean. Tech., 24, 449–462, https://doi.org/10.1175/JTECH1979.1, 2007.
Kazantzidis, A., Bais, A. F., Gröbner, J., Herman, J. R., Kazadzis, S., Krotkov, N., Kyrö, E., den Outer, P. N., Garane, K., Görts, P., Lakkala, K., Meleti, C., Slaper, H., Tax, R. B., Turunen, T., and Zerefos, C. S.: Comparison of satellite-derived UV irradiances with ground-based measurements at four European stations, J. Geophys. Res., 111, D13207, https://doi.org/10.1029/2005JD006672, 2006.
Kouremeti, N., Bais, A., Kazadzis, S., Blumthaler, M., and Schmitt, R.: Charged-couple device spectrograph for direct solar irradiance and sky radiance measurements, Appl. Opt., 47, 1594–1607, https://doi.org/10.1364/AO.47.001594, 2008.
Kripke, M. L.: Antigenicity of Murine Skin Tumors Induced by Ultraviolet Light, J. Natl. Cancer I., 53, 1333–1336, https://doi.org/10.1093/jnci/53.5.1333, 1974.
Krupa, S. V. and Kickert, R. N.: The Greenhouse effect: Impacts of ultraviolet-B (UV-B) radiation, carbon dioxide (CO2), and ozone (O3) on vegetation, Environ. Pollut., 61, 263–393, https://doi.org/10.1016/0269-7491(89)90166-8, 1989.
Lawrence, J. B. and Weir, N. A.: Photodecomposition of polystyrene on long-wave ultraviolet irradiation: A possible mechanism of initiation of photooxidation, J. Polym. Sci. A, 11, 105–118, https://doi.org/10.1002/pol.1973.170110109, 1973.
Mayer, B., Seckmeyer, G., and Kylling, A.: Systematic long-term comparison of spectral UV measurements and UVSPEC modeling results, J. Geophys. Res.-Atmos., 102, 8755–8767, https://doi.org/10.1029/97jd00240, 1997.
Musil, C. F. and Wand, S. J. E.: Responses of sclerophyllous ericaceae to enhanced levels of ultraviolet-B radiation, Environ. Exp. Bot., 33, 233–242, https://doi.org/10.1016/0098-8472(93)90069-R, 1993.
Nevas, S., Gröbner, J., Egli, L., and Blumthaler, M.: Stray light correction of array spectroradiometers for solar UV measurements, Appl. Opt., 53, 4313, https://doi.org/10.1364/ao.53.004313, 2014.
Ogura, R., Sugiyama, M., Nishi, J., and Haramaki, N.: Mechanism of Lipid Radical Formation Following Exposure of Epidermal Homogenate to Ultraviolet Light, J. Invest. Dermatol., 97, 1044–1047, https://doi.org/10.1111/1523-1747.ep12492553, 1991.
Seckmeyer, G., Pissulla, D., Glandorf, M., Henriques, D., Johnsen, B., Webb, A., Siani, A.-M., Bais, A., Kjeldstad, B., Brogniez, C., Lenoble, J., Gardiner, B., Kirsch, P., Koskela, T., Kaurola, J., Uhlmann, B., Slaper, H., den Outer, P., Janouch, M., Werle, P., Gröbner, J., Mayer, B., de la Casiniere, A., Simic, S., and Carvalho, F.: Variability of UV Irradiance in Europe, Photochem. Photobiol., 84, 172–179, https://doi.org/10.1111/j.1751-1097.2007.00216.x, 2008.
Shaw, M. and Goodman, T.: Array-based goniospectroradiometer for measurement of spectral radiant intensity and spectral total flux of light sources, Appl. Opt., 47, 2637–2647, https://doi.org/10.1364/AO.47.002637, 2008.
Sildoja, M. M., Nevas, S., Kouremeti, N., Gröbner, J., Pape, S., Pendsa, S., Sperfeld, P., and Kemus, F.: LED-based UV source for monitoring spectroradiometer properties, Metrologia, 55, S97–SS103, https://doi.org/10.1088/1681-7575/aab639, 2018.
Slaper, H., Reinen, H. A. J. M., Blumthaler, M., Huber, M., and Kuik, F.: Comparing ground-level spectrally resolved solar UV measurements using various instruments: A technique resolving effects of wavelength shift and slit width, Geophys. Res. Lett., 22, 2721–2724, https://doi.org/10.1029/95GL02824, 1995.
Smith, R. C., Baker, K. S., Holm-Hansen, O., and Olson, R.: PHOTOINHIBITION OF PHOTOSYNTHESIS IN NATURAL WATERS*, Photochem. Photobiol., 31, 585–592, https://doi.org/10.1111/j.1751-1097.1980.tb03750.x, 1980.
Sullivan, J. H. and Teramura, A. H.: Effects of Ultraviolet-B Irradiation on Seedling Growth in the Pinaceae, Am. J. Bot., 75, 225–230, https://doi.org/10.1002/j.1537-2197.1988.tb13433.x, 1988.
Teramura, A. H.: Effects of ultraviolet-B irradiances on soybean. I. Importance of photosynthetically active radiation in evaluating ultraviolet-B irradiance effects on soybean and wheat growth, Physiol. Plantarum, 48, 333–339, https://doi.org/10.1111/j.1399-3054.1980.tb03264.x, 1980.
Vaskuri, A., Kärhä, P., Egli, L., Gröbner, J., and Ikonen, E.: Uncertainty analysis of total ozone derived from direct solar irradiance spectra in the presence of unknown spectral deviations, Atmos. Meas. Tech., 11, 3595–3610, https://doi.org/10.5194/amt-11-3595-2018, 2018.
Ylianttila, L., Visuri, R., Huurto, L., and Jokela, K.: Evaluation of a single-monochromator diode array spectroradiometer for sunbed UV-radiation measurements, Photochem. Photobiol., 81, 333–341, https://doi.org/10.1562/2004-06-02-RA-184.1, 2005.
Zerefos, C. S., Tourpali, K., Eleftheratos, K., Kazadzis, S., Meleti, C., Feister, U., Koskela, T., and Heikkilä, A.: Evidence of a possible turning point in solar UV-B over Canada, Europe and Japan, Atmos. Chem. Phys., 12, 2469–2477, https://doi.org/10.5194/acp-12-2469-2012, 2012.
Zong, Y., Brown, S. W., Johnson, B. C., Lykke, K. R., and Ohno, Y.: Simple spectral stray light correction method for array spectroradiometers, Appl. Opt., 45, 1111–1119, https://doi.org/10.1364/AO.45.001111, 2006.
Zuber, R., Sperfeld, P., Riechelmann, S., Nevas, S., Sildoja, M., and Seckmeyer, G.: Adaption of an array spectroradiometer for total ozone column retrieval using direct solar irradiance measurements in the UV spectral range, Atmos. Meas. Tech., 11, 2477–2484, https://doi.org/10.5194/amt-11-2477-2018, 2018a.
Zuber, R., Ribnitzky, M., Tobar, M., Lange, K., Kutscher, D., Schrempf, M., Niedzwiedz, A., and Seckmeyer, G.: Global spectral irradiance array spectroradiometer validation according to WMO, Meas. Sci. Technol., 29, 105801, https://doi.org/10.1088/1361-6501/aada34, 2018b.
Zuber, R., Köhler, U., Egli, L., Ribnitzky, M., Steinbrecht, W., and Gröbner, J.: Total ozone column intercomparison of Brewers, Dobsons, and BTS-Solar at Hohenpeißenberg and Davos in 2019/2020, Atmos. Meas. Tech., 14, 4915–4928, https://doi.org/10.5194/amt-14-4915-2021, 2021.