the Creative Commons Attribution 4.0 License.
the Creative Commons Attribution 4.0 License.
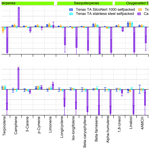
Measurements of atmospheric C10–C15 biogenic volatile organic compounds (BVOCs) with sorbent tubes
Toni Tykkä
Simon Schallhart
Evdokia Stratigou
Thérèse Salameh
Maitane Iturrate-Garcia
Biogenic volatile organic compounds (BVOCs; e.g. terpenes) are highly reactive compounds typically present at sub-parts-per-billion mole fractions in the air. Due to this, their measurements are challenging and they may suffer losses during sampling, storage and analyses. Even though online measurements of BVOCs are becoming more common, the use of sorbent tubes is expected to continue because they offer greater spatial coverage compared to online measurements, and no infrastructure (e.g. electricity, housing/shelter with stable temperature and humidity, sampling lines) is required for sampling. In this study, the performance of an offline technique for the measurement of BVOCs based on sorbent tube sampling was evaluated. Tested compounds included eight monoterpenes, five sesquiterpenes and five oxygenated BVOCs, which are generally either directly emitted (1,8-cineol, linalool, bornyl acetate) or oxidation products (nopinone and 4-acetyl-1-methylcyclohexene). Two sorbent materials (Tenax TA and Carbopack B) and four tube materials (stainless steel (SS), SilcoNert 1000, glass and glass-coated SS) were used. The laboratory evaluations determined the storage stability, breakthrough volumes, suitable tube materials, recovery from ozone scrubbers and particulate filters, and sampling efficiency. In addition, an intercomparison between two laboratories was conducted. No multibed configurations were tested.
Of the sorbent materials Tenax TA showed acceptable results for these BVOCs, while with Carbopack B losses and increases in some compounds were detected. Studied compounds were found to be stable in Tenax TA tubes for at least 1 month at −20 and at +20 ∘C. Breakthrough tests indicated that α- and β-pinene have clearly lower breakthrough volumes in the Tenax TA tubes used (4–7 and 8–26 L, respectively) than other terpenes (> 160 L). SS, SilcoNert 1000 and glass were all shown to be suitable tube materials. Results from Tenax TA sorbent tube sampling agreed with online sampling for most compounds. Heated SS tubes, sodium thiosulfate filters and traps were found to be suitable ozone scrubbers for the studied BVOCs. Tested particle filters had a greater impact on limonene (relative difference < +7 %) than on α- and β-pinene (relative difference ±2 %). The laboratory intercomparison of α- and β-pinene measurements showed that in general, measured values by the two laboratories were in good agreement with Tenax TA.
- Article
(5621 KB) - Full-text XML
-
Supplement
(899 KB) - BibTeX
- EndNote
The global atmospheric burden of biogenic volatile organic compounds (BVOCs) is ∼ 10 times higher than anthropogenic ones (Guenther et al., 2012). The major group of BVOCs in the atmosphere is terpenoids. They are mainly emitted from vegetation (Sindelarova et al., 2014), but in urban areas volatile chemical products, e.g. hygiene and cleaning products, can also be their sources (Hellén et al., 2012a; Steinemann, 2015; Coggon et al., 2021). A recent study by Borbon et al. (2023) indicates that even tailpipe exhausts may be a significant source of terpenoids especially in cities of the developing world. Terpenes are classified according to the number of isoprene (C5H8) units into monoterpenes (C10H16), sesquiterpenes (C15H24) and diterpenes (C20H32). While some forests emit mainly isoprene, boreal forests, for example, are strong mono- and sesquiterpene emitters (e.g. Schallhart et al., 2018; Hakola et al., 2006; 2017; Hellén et al., 2018, 2021).
Terpenes are highly reactive with respect to atmospheric oxidants such as ozone (O3), the hydroxyl radical (OH) and the nitrate radical (NO3), and their atmospheric lifetimes vary from a few minutes to several hours (Acosta Navarro et al., 2014). Terpenes greatly affect the oxidative capacity of the atmosphere and the formation and destruction of ozone. They also participate in the formation and growth of new particles and clouds, impacting the climate. Even small changes in BVOC emissions may substantially modify the radiative properties of clouds (Petäjä et al., 2022). Furthermore, most of the terpenes emitted have a therapeutic potential for inflammatory diseases as a result of their anti-inflammatory effects as well as their function against oxidative stress (Cho et al., 2017; Kim et al., 2020).
Atmospheric mono- and sesquiterpenes have been studied using both direct mass spectrometer (MS) and chromatographic methods. Proton transfer reaction mass spectrometers (PTR-MSs), both quadrupole and time-of-flight (TOF), have been used for measurements of monoterpene ambient air amount fractions (e.g. Peräkylä et al., 2014) and emissions (e.g. Schallhart et al., 2018). More recently Vocus PTR-TOF instruments have also been used for measurements of sesquiterpenes (Li et al., 2020, 2021). With these direct mass spectrometric techniques, high time resolution (even seconds) can be achieved, but they lack the species-specific information and all monoterpenes or sesquiterpenes are detected as a sum. Due to the variable reactivities of terpenes with respect to atmospheric oxidants, detailed information is needed for estimating their atmospheric impacts.
In addition to these direct MS methods, in situ chromatographic methods, where the sample is introduced directly into a thermal desorption unit of an analysing instrument, have been used for species-specific detection of BVOCs of ambient air (e.g. Bouvier-Brown et al., 2009; Hakola et al., 2012; Hellén et al., 2012a, 2018, 2020; Yee et al., 2018; Mermet et al., 2019, 2021) and emissions (e.g. Hakola et al., 2017; Hellén et al., 2020, 2021). These in situ methods need good infrastructures with stable room temperature and electricity. Therefore, sorbent tubes, which can be easily transported to several locations with low or no infrastructure, are also used. With sorbent tubes, one analytical instrument in the laboratory can be used for analysing samples from several locations. The tubes are also re-usable. Therefore, sorbent tube sampling is a cost-effective alternative to BVOC sampling, especially in locations where in situ measurements are not possible. However, the time resolution of sorbent tubes is often poor and sampling is more laborious compared to in situ “online” measurements.
Sorbent tubes are commonly used for offline sampling of VOCs in the air, for both ambient air and emission studies. While there are standard and reference methods available for sorbent tube sampling of aromatic hydrocarbons (e.g. ISO/DIS 16017-2, 2003 and CEN, 2005) emitted by the different anthropogenic sources (e.g. traffic and biomass burning), much less knowledge is available on their suitability for different BVOCs. The most common sorbent, used in the ambient air studies of terpenes, has been Tenax TA (e.g. Hakola et al., 2003; Jardine et al., 2015; Zannoni et al., 2016). In addition, carbon-based sorbents, e.g. Carbotrap (Gallego et al., 2010), Carbopack B (Oh et al., 2010), Carbograph 1 and Carbograph 5 (Yáñez-Serrano et al., 2018; Song et al., 2012), and Chromosorb 106 (Sunesson et al., 1999), have been used in ambient air, lab or emission studies. Often Tenax TA is used together with other stronger sorbents to widen the selection of the compounds, which can be measured quantitatively (e.g. Helin et al., 2020; Jardine et al., 2015; Hakola et al., 2003; Zannoni et al., 2016).
There are several different types of sorbents and tube materials available, and their suitability depends greatly on the studied compounds. Therefore, suitability of materials used should be evaluated for all compounds of interest. Tests with sorbent tubes for analysis of some monoterpenes (α-pinene, β-pinene and limonene) have been conducted by, for example, Komenda et al. (2001), Arnts (2010), Gallego et al. (2010), Ullah and Kim (2014), Ahn et al. (2016), and Sheu et al. (2018), but the performance of the tubes may vary greatly even between different monoterpenes and very little information is available on their suitability for sesquiterpene or C10–C15 oxygenated BVOC measurements (Helmig et al., 2004; Helin et al., 2020).
Since most of the studied BVOCs are highly reactive with ozone (O3) and can also be oxidized during sampling on the sorbent tubes, ozone scrubbers are used to remove ozone in front of them. Several different O3 removal techniques have been used in VOC measurements, e.g. MnO2 nets, sodium-thiosulfate-impregnated filters, heated stainless-steel (SS) tubes, copper tubes coated with potassium iodide and NO titration (Helmig, 1997; Fick et al., 2001; Pollmann et al., 2005; Hellén et al., 2012b). Some of the O3 scrubbers are known to have very short lifetimes (Fick et al., 2001; Bouvier-Brown et al., 2009), while some may suffer losses of the most reactive compounds (Calogirou et al., 1996; Pollmann et al., 2005). In addition to the scrubber material or type, optimizing size and flow is also critical for achieving sufficient O3 removal without losing the studied BVOCs (Hellén et al., 2012b).
Filters are used to remove particulate matter in sorbent tube measurements and analyses. Particle filters are recommended to avoid any contamination of all the fluidic parts of a measurement system, especially the more sensitive ones such as valves, measurement cells, preconcentration traps and reactors. Guidelines mostly recommend polytetrafluoroethylene (PTFE) or perfluoroalkoxy (PFA) membranes as well as stainless-steel screens as particle filters (e.g. Steinbrecher and Weiß, 2012; Reimann et al., 2018). VOCs are not trapped by these filters, but they may suffer some losses.
Here the suitability of sorbent tubes for C10–C15 BVOC measurements was evaluated in the framework of the EMPIR (European Metrology Programme for Innovation and Research) project Metrology for Climate Relevant Volatile Organic Compounds (MetClimVOC; https://www.metclimvoc.eu/, last access: 12 December 2023). Tested compounds included eight monoterpenes, five sesquiterpenes and five oxygenated BVOCs, which are generally either directly emitted (1,8-cineol, linalool, bornyl acetate) or oxidation products (nopinone and 4-acetyl-1-methylcyclohexene). The laboratory evaluations determined storage stability, breakthrough volumes, suitable tube materials, recoveries from O3 scrubbers and particulate filters, and the sampling efficiency. In addition, an intercomparison between two laboratories was conducted. Based on earlier BVOC studies, two sorbents (Tenax TA and Carbopack B, mesh) were selected for the laboratory evaluations.
2.1 Chemicals and materials
Methanol (≥ 99.9 %) for producing BVOC standards was purchased from VWR International (Gliwice, Poland), and pure solutions of the studied compounds (listed in Table 1) were purchased from Sigma-Aldrich (St. Louis, MO, USA). Tested compounds included eight monoterpenes (α-pinene, camphene, myrcene, β-pinene, Δ3-carene, p-cymene, limonene, terpinolene), five sesquiterpenes (longicyclene, isolongifolene, β-farnesene, β-caryophyllene, α-humulene), five oxygenated BVOCs (1,8-cineol, linalool, 4-acetyl-1-methylcyclohexene (4AMCH), nopinone and bornyl acetate) and three aromatic hydrocarbons (toluene, o-xylene and 1,3,5-trimethylbenzene) for the comparison. For producing standard solutions, ∼ 30 mg of each BVOC was added to the 500 mL of methanol, which was further diluted into six different amount-of-substance fractions (a.k.a. amount fractions). For producing VOC-rich air, a methanol solution containing 10–15 µg mL−1 of studied terpenes was injected into zero air via a Teflon PTFE T piece using an automatic syringe pump (at an injection flow rate of 6–15 µL h−1). Zero air with a flow of 1–2 L min−1 used in the tests was generated using a zero-air generator (HPZA-7000, Parker Balston, Lancaster, NY, USA). The air was humidified to the desired level by bubbling a fraction of air through the ultrapure water (Milli-Q Gradient, Molsheim, France).
Table 1List of the studied compounds with limits of quantification for Tenax TA (LOQT) and Carbopack B (LOQC) tubes and uncertainty for Tenax TA (UT) and Carbopack B (UC) tubes. LOQ and U (k=2) were calculated for the sampling volume of 1.5 L and amount of ∼ 30 ng of each studied compound. The equations for calculating uncertainties can be found in Sect. S1 in the Supplement.
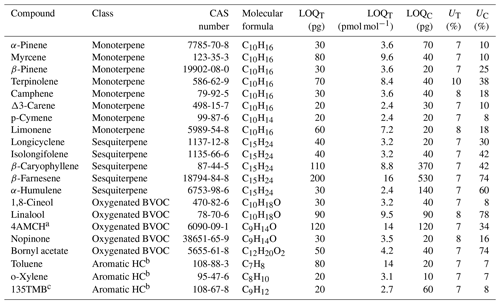
a 4AMCH denotes 4-acetyl-1-methylcyclohexene. b HC denotes hydrocarbon. c 135TMB denotes 1,3,5-trimethylbenzene.
The tested tubes consisted of commercial Tenax TA (), self-packed Tenax TA () and commercial Carbopack B (; see Table S1 in the Supplement for more details). The tubes were packed following the instructions by PerkinElmer Inc. (2007). Carbopack B has also been used in cold traps of online thermal desorption–gas chromatography–mass spectrometers (TD-GC-MSs) (e.g. Mermet et al., 2019) and in multibed sorbent tubes together with Tenax TA (e.g. Ullah and Kim 2014; Iqbal et al., 2014; Helin et al., 2020). The main tube material was stainless steel (SS), which was also the most common material in earlier studies. In addition, a set of self-packed SilcoNert 1000 tubes with Tenax TA were used. Even though SilcoNert 1000 is not a commonly used material in sorbent tube sampling, it is known to be a more inert material compared to SS, and its use could improve the results.
The self-packed sorbent tubes were prepared by packing empty stainless-steel or SilcoNert 1000 tubes (PerkinElmer Inc., Waltham, MA, USA) with Tenax TA () purchased from Sigma-Aldrich (St. Louis, MO, USA). Silanized glass wool (Phase Separations Ltd., Deeside, UK), stainless-steel mesh (Markes International, Llantrisant, UK) and a gauze-retaining spring (Markes International, Llantrisant, UK) were used to prevent sorbent phase mixing and exiting.
Before the sampling, the sorbent tubes used were re-conditioned by heating to 320 ∘C with 50 mL min−1 flow of helium for 15 min using a thermal desorption unit (TurboMatrix 350, PerkinElmer). Sampling was conducted using a pump (N 86 Laboport, KNF).
2.2 Analysis
The sorbent tubes from the BVOC tests were analysed using a thermal desorption unit (TD unit, TurboMatrix 350, PerkinElmer) connected to a gas chromatography (GC) instrument (Clarus 680, PerkinElmer) coupled to a mass spectrometer (MS, Clarus SQ 8T, PerkinElmer). These tubes were desorbed in a helium flow of 50 mL min−1 at 300 ∘C for 5 min. The sample was focused into a Tenax TA cold trap kept at 20 ∘C. From there, the sample was transferred to the GC instrument through a heated transfer line by rapid heating up to 300 ∘C in a helium flow. A DB-5 column (length 60 m, internal diameter (i.d.) 0.25 mm, film thickness 1 µm, from Agilent Technologies) was used for separation. The GC column oven temperature was initially set at 50 ∘C, from which it was increased to 150 ∘C at a rate of 4 ∘C min−1 and then at a rate of 8 ∘C min−1 to 280 ∘C, where it was kept for 8.75 min. The helium carrier gas flow was 1 mL min−1. An online mode air sample was taken directly into the cold trap of the TD-GC-MS without first focusing it into a sorbent tube. This method has been described in Helin et al. (2020). The TD-GC-MS was calibrated using methanol solutions for BVOCs. Methanol solutions (5 µL) of the studied VOCs were injected into the sorbent tubes using a flow of ∼ 80 mL min−1 of nitrogen with a quality of 99.9999 % (50 L cylinder, Linde Gas). Methanol was flushed away for 10 min. The final tubes contained 0.25–78.9 ng of the studied compounds depending on the calibration level. Six-level calibration was used. Both Tenax TA tubes and multibed tubes with Tenax TA and Carbopack B were used for calibrating the instrument. Repeatability of the calibration for the studied compounds was 2 %–4 %, being the highest percentage for terpinolene. For the comparison of calibration methods, a gaseous standard from the National Physical Laboratory (NPL, UK, date of issue 14 October 2020) containing 2 nmol mol−1 α-pinene, Δ3-carene, 1,8-cineol and limonene was used with a dual-stage regulator (R200/2-6SS, Linde plc). The gaseous standard was sampled both into the sorbents of the tubes and directly to the cold trap of the TD unit. Sorbent tubes were sampled from the outlet of the gas cylinder valve using a T connector and a pump (N 86 Laboport, KNF) with the sampling flow of ∼ 100 mL min−1. The main standard gas flow was kept at ∼ 200–500 mL min−1 during sampling. For the direct measurements fluorinated ethylene propylene (FEP) tubing (length ∼ 0.5 m, i.d. in.) and flow of 40 mL min−1 was used. The sampling time was 10 min for both methods.
The blanks were below the detection limit for all studied compounds except toluene. For toluene, blank amounts of 0.47 and 0.22 ng were found in the Tenax TA and Carbopack B tubes, respectively. The quantification limits presented in Table 1 were calculated as 10 times the signal-to-noise ratio, and they varied between 20 and 200 pg per tube.
The uncertainty was estimated for the sampling volume of 1.5 L and amount of ∼ 30 ng of each studied compound. The calculation of the total uncertainty is described in detail in Sect. S2. The uncertainty in the studied compounds in the Tenax TA and Carbopack B tubes was 7 %–10 % and 7 %–78 %, respectively (Table 1).
An additional TD-GC flame ionization detector (FID) was used for particulate filter studies. Samples entered the instrument through a preconcentrator provided with three traps. In a first step of the preconcentration, samples passed through a cold multisorbent trap (Tenax TA and glass beads) at −135 ∘C to eliminate the water in the sample. Then the trap was heated to 120 ∘C and the sample passed along a second additional cold trap of Tenax TA at −55 ∘C, where it was cooled and then heated to 180 ∘C to remove the CO2 present in the samples. Finally, the sample reached a third trap where a flow of liquid nitrogen at −200 ∘C passed, and then the sample was heated to 150 ∘C. The compounds were desorbed and injected into the GC instrument for separation and analysis with the FID. The separation was performed using a dual-capillary-column system of (39 m × 0.32 mm × 5 µm) for C2–C5 hydrocarbons and CP-Sil 5 CB (50 m × 0.32 mm × 1.2 µm) for C6–C10 hydrocarbons. The calibration of the TD-GC-FID was performed with an NPL calibration standard containing α-pinene, β-pinene, limonene and toluene at around 4 nmol mol−1 for at least five replicates to assure a good repeatability. The coefficients of variation were below 2 %.
2.3 Storage stability tests
The storage stability was tested by injecting known amounts (25–39 ng) of the studied compounds into the sorbent tubes as methanol solutions similarly to the preparation for the calibration tubes. Excess methanol was flushed away for 10 min in nitrogen (99.9999 %) flow of ∼ 80 mL min−1 before the storage and analyses. The tubes were sealed with brass Swagelok caps and PTFE ferrules. One-third of the tubes (i.e. four commercial and five self-packed SS Tenax TA, three self-packed SilcoNert 1000 Tenax TA and five commercial Carbopack B) were analysed immediately, one-third were kept at room temperature (∼ 22 ∘C) for 1 month and one-third were kept at −20 ∘C for 1 month before the analysis.
The relative difference in the BVOCs in the sorbent tubes kept at (a) +22 ∘C and (b) −20 ∘C for 1 month compared to the tubes analysed immediately was calculated using Eq. (1):
where mi is the mass (ng) of studied compounds in the tubes after storage period of i and m0 is the mass (ng) of studied compound in the tubes analysed immediately after the preparation.
2.4 Breakthrough volumes
To determine safe sampling/breakthrough volumes of BVOCs, breakthrough tests were conducted. BVOCs were injected into the zero airflow to produce BVOC-rich air. Most of the tests were conducted with methanol solutions of BVOCs injected into the zero air, producing amount fractions of 0.1–0.9 nmol mol −1. However, two additional tests were conducted for α- and β-pinene using a portable generator of reference gas mixtures (ReGaS2; Pascale et al., 2017; see Sect. S1 for more details) – based on the permeation method (ISO 6145-10, 2002) – to check if methanol injected with BVOCs into the zero air affected the breakthrough volumes. In ReGaS2 tests, amount fractions of ∼ 10 nmol mol−1 were used.
Sorbent tubes were attached onto the inlet of the instrument, and the amount fraction levels breaking through the tubes were followed online with the TD-GC-MS. Breakthrough (B) was calculated following Eq. (2):
where Xin is the amount fraction of VOCs in the air flushed into the tube and Xout is the amount fraction in the air coming out from the tube.
Breakthrough volumes were defined as a volume when 5 % of the injected amount fraction passes through the tube i.e. the volume when B=5 % (CEN, 2005).
2.5 Sampling efficiency
To study the suitability of the calibration method used, a comparison with reference gas from NPL containing 2 nmol mol−1 α-pinene, Δ3-carene, 1,8-cineol and limonene was conducted by calibrating the instrument with methanol solution and analysing the reference gas with the online mode of the instrument. Three sampling times of 10, 20 and 30 min were used with a flow of 40 mL min−1. The expected sample masses were 4.3–4.7, 8.5–9.3 and 12.8–14 ng for 10, 20 and 30 min sampling, respectively. Three replicate samples were taken with each sampling time.
The relative differences between the amount fractions of the studied compounds in the sorbent tubes compared to the expected amount fractions were calculated using Eq. (3):
where mj is the mass (ng) of studied compound in the tubes (j) and me is the expected mass (ng).
Linearity of the response related to the sampling amount was used as one indication of the sampling efficiency. The sampling efficiency of the sorbent tubes was also studied by comparison with online TD-GC-MS results. The studied compounds were injected as methanol solutions into the zero airflow, and the amount fraction levels were measured in parallel with different sorbent tubes and an online TD-GC-MS. Both commercial and self-packed SS and SilcoNert 1000 Tenax TA tubes as well as commercial Carbopack B tubes were tested. The sampling efficiency of the sorbent tubes was estimated by sampling different amounts/volumes and by comparing the results to online TD-GC-MS results. The flow used for sorbent tube sampling was ∼ 200 mL min−1. Tests were conducted at two different relative humidities (RH ∼ 30 % and ∼ 70 %). The air was humidified to the desired level by bubbling a fraction of air through ultrapure water (Milli-Q Gradient, Molsheim, France). RH was measured with a Vaisala HMI33 device (probe HMP35, Vaisala, Helsinki, Finland). The sampled volumes for tubes were 3, 6 and 12 L and for the online sampling were 0.6, 1.2 and 2.4 L. Sampled amounts were 2–80 ng per tube. The recovery of the sorbent tubes was compared to the recovery of the online sampling.
An additional test of linearity was conducted, using only commercial Tenax TA tubes, by taking five different sample volumes (0.4, 0.6, 0.8, 1.0 and 1.2 L) from the ReGaS2 outlet. Amount fractions obtained by sampling different volumes were compared. The sampled amount of α-pinene in the tubes varied from 14 to 44 ng. The commercial Tenax TA tubes were also compared with online TD-GC-MS while sampling from the ReGaS2 permeator. Both tubes and online samples were taken for 15 min with a flow of 40 mL min−1 (sample volume 0.6 L).
Additional sampling efficiency tests were conducted for α-pinene, Δ3-carene, 1,8-cineol and limonene with the 2 nmol mol−1 reference standard gas (NPL, UK). Samples were taken from the gas into the commercial and self-packed Tenax TA and commercial Carbopack B tubes with a sampling flow of ∼ 100 mL min−1 and sampling time of 10 min. Three replicates were taken of each type of tube. Samples were analysed with the TD-GC-MS using the calibration with methanol solutions, which was also used in the other experiments, and detected amounts were compared to expected amounts. At the same time, samples were taken using the online mode of the TD-GC-MS.
2.6 Tests with different tube materials
To study the impact of the sorbent tube materials on the recoveries, BVOC-rich air was flushed through empty sorbent tubes made of SS, glass-coated SS, glass and SilcoNert 1000, and the relative difference from the situation where an empty tube was not used in front of the sorbent tube was followed (Eq. 4, Fig. 1). To produce terpene-rich air for the test, studied compounds were injected as methanol solutions into the zero airflow. Sorbent tube samples were taken from the terpene-rich zero airflow with (sorbent tube 2) and without (sorbent tube 1) an empty tube attached in front (Fig. 1). The sampling time was 30 min, and the flow was 100 mL min−1. The sampled masses of the individual compounds were ∼ 14–23 ng per tube. The relative difference between the masses found in the sorbent tubes 1 (m1) and 2 (m2) was calculated following Eq. (4):
2.7 O3 scrubber tests
To determine the effect of different O3 scrubbers on amount fractions of the studied BVOCs, methanol solutions of them were injected into a zero airflow and measured upstream and downstream of the scrubbers with and without ozone. Four O3 scrubber types were used; a heated stainless-steel tube (heated SS) at 120 ∘C, a filter impregnated with sodium thiosulfate (Na2SO3), manganese dioxide (MnO2) net and a copper tube coated with potassium iodide (). Table 2 presents the details of the scrubbers used. The ozone level used was ∼ 40 nmol mol−1. Flows through the scrubbers were set at 1.0, 0.1, 0.08 and 0.1 L min−1 for , MnO2, Na2SO4 and heated SS scrubbers, respectively. New and aged scrubbers were tested. This procedure was performed at relative humidity values of 30 %–36 % and at room air temperature (∼ 22 ∘C). A detailed schematic of the set-up is depicted in Fig. S1 in the Supplement.
Sorbent tube (Tenax TA /Carbopack B ) sampling was conducted with a flow of ∼ 80 mL min−1 and a sampling time of 15–60 min. Simultaneous samples were always taken upstream and downstream of the scrubbers. The relative difference (Rel.diffo) of the masses found in the samples with (mw) and without (mwo) the O3 scrubbers was calculated from Eq. (5):
2.8 Tests for particulate filters
Three types of particle filters were tested – Balston filters, PTFE membranes and SS screens (Table 3) – to evaluate possible BVOC losses on filters commonly used in their measurements and analyses. Experiments were performed using an NPL gaseous standard cylinder containing α-pinene, β-pinene, limonene and toluene. Measurements with and without the particle filters were performed at a sampling flow rate of 0.100 L min−1 and at 65 %–75 % relative humidity. The measured amount fractions were ∼ 1 nmol mol−1. While other studies were conducted using a TD-GC-MS, these experiments were conducted using a thermal desorption unit connected to a TD-GC-FID.
For the blanks, we conducted at least three injections of zero air into the TD-GC-FID to verify the background of the zero air, and subsequently the filters were inserted into the inlet of the system to verify the background of each filter. Then the targeted compounds were inserted into the system, and measurements with and without the filter were conducted for three to four repetitions. Figure S2 shows the set-up of these experiments.
After considering the zeros and calculating the net normalized signal (NET , where M is the measured quantity), the relative differences (Eq. 6) between the value measured upstream of the particle filter (MWOF) and the value measured downstream of the filter (MWF) were calculated.
2.9 Laboratory intercomparison
To evaluate the performance of the sorbent tubes under study, the Finnish Meteorological Institute (FMI) and IMT Nord Europe conducted an interlaboratory comparison. For that purpose, IMT Nord Europe loaded 16 Tenax TA (mesh ) and 16 Carbopack B (mesh ) SS tubes with α-pinene, β-pinene and toluene using ReGaS2. The loading flow was 50 mL min−1 for each tube. Half of the tubes of each sorbent material were loaded in 15 min and the other half in 30 min (see Sect. S1). Four sorbent tubes of each sorbent type were not loaded (i.e. blanks). The blanks were transported together with the sampling tubes to assess potential contamination during the transport. Each laboratory analysed half of the tubes (including blanks).
FMI analysed the tubes within 2 weeks of sampling using the TD-GC-MS methods described in this study, and IMT Nord Europe performed the analysis immediately after sampling with a TD (TurboMatrix 350, PerkinElmer, USA)-GC instrument connected to a flame ionization detector (FID) (Clarus 680, PerkinElmer) and a mass spectrometer (MS) (Clarus SQ 8T, PerkinElmer). The calibration of the TD-GC-FID/MS was performed with an NPL (UK, date of issue 20 March 2020) calibration standard containing non-methane hydrocarbons (NMHCs) from C2 to C9 (including toluene) and three monoterpenes (α-pinene, β-pinene, limonene) at around 4 nmol mol−1. In order to cover the range of the amount fractions, we have established the linearity curve with different sampling duration (15, 30 and 60 min) while maintaining the same sampling flow (21 mL min−1). The analytical method used by IMT Nord Europe has been described in detail by Debevec et al. (2021) and references therein.
3.1 Storage stability
The National Institute for Occupational Health and Safety (NIOSH, USA) recommends that if the average quantitative measurements of the samplers differ from the set analysed on day 0 by more than 10 %, the method in question does not meet the sample storage stability criterion (Kennedy et al., 1996). Here 1-month storage stability in sorbent tubes was tested. For that purpose, both self-packed and commercial Tenax TA tubes and commercial Carbopack B tubes were used.
All studied compounds were relatively stable for 1 month in all tested Tenax TA tubes stored at both −20 and +22 ∘C (Fig. 2). The mean relative differences in the Tenax TA tubes kept at −20 and at +22 ∘C for 1 month compared to tubes analysed immediately were −7 % to +13 % and −16 % to +16 %, respectively. These differences indicated that storage in a freezer may slightly improve the storage stability. No clear differences were detected between the SilcoNert 1000 and SS tubes or between self-packed and commercial tubes. Additionally, in earlier studies many monoterpenes have been found to be stable in Tenax TA or in multibed sorbents containing Tenax TA for at least 14–62 d (Sunesson et al., 1999; Sheu et al., 2018; Helin et al., 2020). α-Pinene was stable even after 12 months of storage (Demichelis et al., 2009). However, Volden et al. (2005) had a recovery of only 69 %–93 % for α- and β-pinene in Tenax TA after storage of 7, 14 and 28 d at 5 and 20 ∘C. Earlier data on other BVOCs are more limited. Helin et al. (2020) studied several mono- and sesquiterpenes in Tenax TA – Carbopack B multibed sorbents and monoterpenes were recovered at 101±2 %, 93±5 % and 97±4 % after 5 d, 1 month and 2 months of storage at 4 ∘C, respectively. Similarly, sesquiterpenes were recovered on average at 104±2 %, 89±3 % and 94±5 % after 5 d, 1 month and 2 months of storage, respectively.
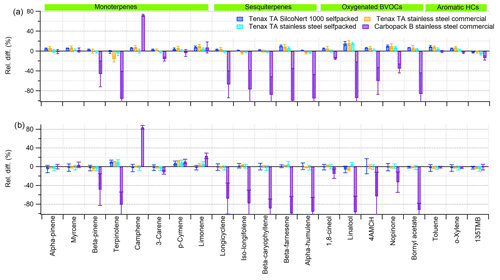
Figure 2Relative difference in the sorbent tubes kept at (a) +22 ∘C and (b) −20 ∘C for 1 month compared to the tubes analysed immediately. Error bars show the standard deviation (1σ) of the replicates (N= 4, 5, 3 and 5 for Tenax TA stainless-steel commercial, Tenax TA stainless-steel self-packed, Tenax TA SilcoNert 1000 self-packed and Carbopack B commercial, respectively).
In Carbopack B tubes there were significant losses of most terpenes and only α-pinene, myrcene, p-cymene and 1,8-cineol had 100±10 % recovery at both temperatures (Fig. 2). Even though it would be expected that the compounds would be more stable at −20 relative to +20 ∘C, sometimes even larger deviations were observed in Carbopack B at −20 ∘C. Some deviations were also detected in the tubes analysed immediately, possibly for example due to incomplete desorption or reactions on the sorbent surface. The recovery of camphene was clearly higher than expected (> 170 %), which indicates that the isomerization of terpenes is occurring in the tubes. It is also possible that some of the terpenes (especially sesquiterpenes) are not fully desorbed from the tubes during the analysis. For aromatic hydrocarbons the results were also good for the Carbopack B tubes, which was expected based on earlier studies (e.g. Hellén et al., 2002).
3.2 Breakthrough volume
Camphene and α- and β-pinenes had the lowest breakthrough volumes of the studied compounds. The breakthrough volumes of camphene and α- and β-pinenes in the Tenax TA tubes were 4–16, 5–16 and 8–26 L, respectively (Table 4). Some breakthrough was detected also for Δ3-carene, limonene and 1,8-cineol with breakthrough volumes of 33–156, 38–158 and 16–76 L, respectively. For the self-packed tubes, the volumes were lower than for the commercial ones. For the commercial tubes, breakthrough volumes for RH 70 % were lower than for RH 30 %. The impact of RH was not detected for the self-packed tubes. The retention of the compounds on the sorbent is based on the competition of the analyte with other molecules in air. Even though Tenax TA is a hydrophobic sorbent at high humidity, some water may still be adsorbed, or water may condense onto the tube, impacting the sorption of other compounds. In earlier studies the impact of RH on Tenax TA retention was not clear since often Tenax TA was studied in multibed sorbents. In their breath sample studies, Wilkinson et al. (2020) found that high relative humidity during sampling generally reduces the ability of Tenax TA/Carbograph sorbent tubes to capture volatile compounds. However, since the impact was more pronounced for the less volatile compounds with higher breakthrough volumes, they speculated that impact is other than breakthrough. Ho et al. (2017) found significant impacts of RH on retention of C2–C5 aliphatic hydrocarbons in multisorbent (Tenax TA, Carbograph 1 TD, Carboxen 1003) tubes. The breakthrough volumes for C2 aliphatic compounds were reduced by 92 %–95 % under 90 % RH, and the main impact was expected to be through the less hydrophobic sorbent Carboxen 1003. For > C5 compounds, no breakthrough was detected by Ho et al. (2017). In the studies of Maceira et al. (2017), humidity problems were demonstrated with carbon-based tubes, while Tenax-based tubes did not display any influence. Our tests with commercial tubes at 30 % humidity were done for the totally new tubes, and this could also explain the higher retention. In the tests under 70 % humidity conducted after the tests of 30 % humidity, any difference between commercial and self-packed tubes was not that clear. However, tests conducted later with a ReGaS2 generator with commercial tubes at RH 30% show slightly higher breakthrough volumes, albeit not as high as for the first tests (Fig. 3), indicating some influence of RH. Increasing the temperature of the sorbent tube to 10 ∘C above the temperature of the air sample could reduce the interference of the humidity (Karbiwnyk et al., 2002). Also, amount fractions used for the commercial tubes in the tests with methanol solutions that were a bit lower may have had an impact (Table 4).
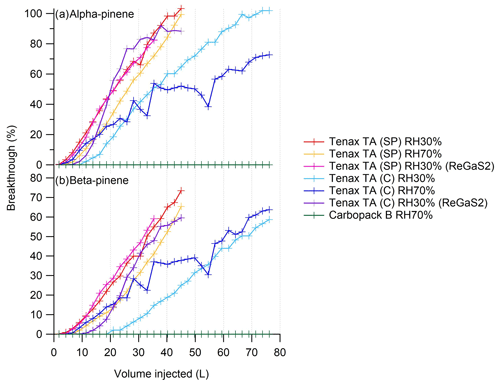
Figure 3Breakthrough of (a) α-pinene and (b) β-pinene from Tenax TA and Carbopack B sorbent tubes (SP, self-packed tube; C, commercial) at relative humidity of 30 % and 70 %. Monoterpenes were either injected into the zero air as methanol solutions or generated by the portable generator (ReGaS2).
Table 4Breakthrough volumes (V_brkt, 5 %) of BVOCs measured by injecting methanol solutions of studied compounds into zero air. Values in parentheses are the amount fractions (nmol mol−1) of the studied BVOCs in the sampled air.
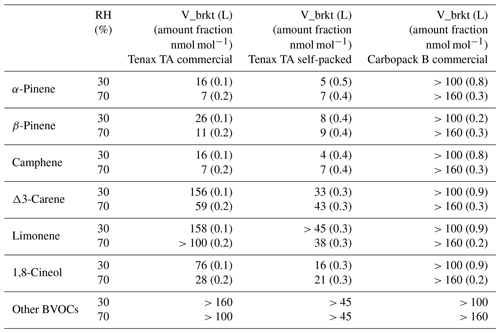
No breakthrough was detected for the other BVOCs in Tenax TA tubes even with a sampling volume of 160 L. There was no breakthrough for the studied compound through the Carbopack B tubes. Results for α- and β-pinenes from permeator tests were similar to those with methanol injection (Fig. 3), and, therefore, methanol used as solvent was not expected to have significant effect on the breakthrough volumes.
Estimates of breakthrough volumes can be found in the literature, but most earlier studies have included only α-pinene, β-pinene and/or limonene (Arnts, 2010; Gallego et al., 2010; Sheu et al., 2018), while in our study several BVOCs were tested. These earlier results are in accordance with our findings on α- and β-pinene having lower safe sampling volumes in Tenax TA (4–7 L) than limonene (≥ 38 L). In addition to this, our study found that other C10–C15 BVOCs also have higher safe sampling volumes, except camphene, which was breaking through at the same volumes as α- and β-pinene. In studies on Tenax TA in multibed tubes together with stronger carbon-based sorbents, much higher breakthrough volumes (even > 24 L) were detected even for α- and β-pinene (Veenas et al., 2020; Helin et al., 2020; Komenda et al., 2001). Additionally, this is in accordance with our results on breakthrough volumes in Carbopack B tubes being very high (> 160 L) for all studied compounds. Due to lower amount of each sorbent in multibed tubes, especially α- and β-pinene may be breaking through the Tenax TA into the carbon-based sorbent at very low sampling volumes. The behaviour of the compounds in these carbon sorbents should also be considered to be shown by losses and possible isomerization of some terpenes (e.g. β-pinene) in Carbopack B in this study.
3.3 Sampling efficiency
From the comparison between the NPL gas standard and the methanol solution calibration methods, relative differences in other compounds were within ±10 % criteria, but for limonene a slightly greater (−20 % to −15 %) difference from the expected amount fraction of the NPL reference gas was detected (Fig. 4a). All three different sampling times showed similar recoveries. However, with an increased sampling time the standard deviation between the replicates clearly decreased.
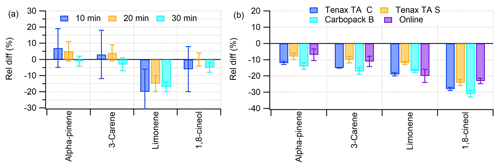
Figure 4(a) Relative difference between the measured and expected amount of NPL gas standard with different online mode sampling times. (b) Relative difference between the measured and expected amount of NPL gas standard flushed into different tubes (C, commercial; S, self-packed) or analysed with the online mode of the instrument (online). Error bars show the standard deviation between the three replicate samples. For both tests the instrument was calibrated with methanol solutions of BVOCs.
For most of the studied BVOCs, Tenax TA tubes showed good linearity with the sampling volumes (3, 6 and 12 L) and sampled amounts (2–80 ng) used. Examples of the linearity fits can be found in Fig. S2. Decreased amount fractions (∼ 25 % lower than expected) were detected for α- and β-pinene and camphene with self-packed tubes with the highest sampling volume of 12 L. This is in accordance with the breakthrough volume results where the breakthrough volume of camphene and α- and β-pinene was 4, 4, and 8 L, respectively. In addition, it was not possible to verify the linearity of sesquiterpenes due to instabilities in production of sesquiterpene-rich air. Comparisons between the tubes and online samples taken at the same time periods were still possible. Especially linalool and β-farnesene had higher yields in Tenax TA sorbent tubes compared to online sampling (Fig. 5). In earlier studies, these compounds have been found to have losses in online sampling systems (Helin et al., 2020).
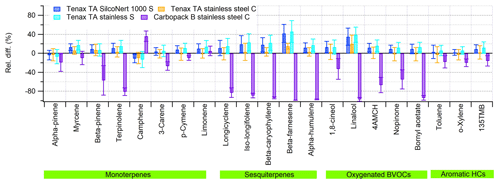
Figure 5Relative difference (%) of the sorbent tubes (C, commercial; S, self-packed) compared to the online sampling results, i.e. the ratio of the difference between the sorbent tube and online sampling amount fractions to the online amount fractions. The relative humidity of the sampling air was ∼ 70 %. Error bars show the standard deviation of the three replicate tubes taken with each sorbent sampling volume (3, 6 and 12 L).
No clear difference between SilcoNert 1000 and SS tubes was detected in the linearity tests or in comparison with online samples (Figs. 5 and S2). The mean standard deviation of the six sets of parallel SilcoNert 1000 and SS tube samples was 4 %. Tests were conducted at relative humidity of 30 % and 70 %. The humidity of the sampling air did not influence the results.
In Carbopack B tubes most of the compounds had severe losses at all sampling volumes (Fig. 5). At the same time, the amount fraction of some terpenes (e.g. camphene) increased. This indicates that some terpenes can be isomerized, forming other terpenes in the Carbopack B tubes.
In the additional test using ReGaS2, where sampled amounts of α-pinene varied from 14 to 44 ng, high linearity was observed. The standard deviation between the amount fractions measured with different sampling volumes was 1.2 %. For β-pinene the amount fractions produced by ReGaS2 were out of the calibration range of the TD-GC-MS. The amounts of β-pinene collected varied from 40 to 103 ng, while the highest calibration point was 69 ng. Possibly due to the exceeded calibration range, the amount fractions measured at higher volumes of 1.0 L (88 ng) and 1.2 L (103 ng) were 11 % and 16 % lower, respectively, than the amount fraction measured with only 0.4 L (40 ng).
The commercial Tenax TA tubes were also compared with the online TD-GC-MS while sampling from ReGaS2. Both tubes and online samples were taken for 15 min with a flow of 40 mL min−1. The α-pinene, β-pinene and myrcene amount fractions in the tubes were 95 %, 100 % and 120 % of the online amount fractions, respectively.
In the sampling efficiency tests using the NPL gas standard, slightly lower than expected amounts were measured, especially for 1,8-cineol with both sorbent tubes and the online TD-GC-MS (Fig. 4b). For sorbent tubes the relative differences from the expected amounts of α-pinene, Δ3-carene, limonene and 1,8-cineol were −12 % to −8 %, −17 % to −10 %, −19 % to −12 % and −31 % to −24 %, respectively. For the online TD-GC-MS, the relative differences to the expected amounts of α-pinene, Δ3-carene, limonene and 1,8-cineol were −7 %, −11 %, −20 % and −23 %, respectively.
3.4 Impact of tube material
The results show that the relative differences with different tube materials were between −15 % and +20 % for most compounds. This is expected to be within uncertainty in this test set-up. Higher-than-average uncertainty (Table 1) was caused by the fluctuations in sampling flows and drifts of the MS response. However, there were clear losses of some sesquiterpenes (β-caryophyllene, β-farnesene and α-humulene) and oxygenated BVOCs (linalool and 4AMCH) on SS tubes (Fig. 6). For these SS tubes slightly higher amounts of p-cymene and limonene were also detected.
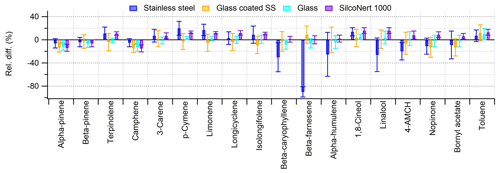
Figure 6Relative difference in the studied BVOCs flushed through the empty tubes (SS, stainless steel) compared to the no-tube-used situation (see Fig. 1 for the test set-up).
Earlier studies by Helin et al. (2020) showed significant losses of β-farnesene and α-humulene, as well as some oxygenated sesquiterpenes and diterpenes, when using empty SS tubes. In their studies, the recoveries were significantly improved using glass tubes. SilcoNert 1000, which was found in this study to be a suitable material too, was not tested in the earlier study. When the tubes are filled with sorbent material, the tube surface is much smaller, and therefore the sample has less contact with the tube walls and the losses are expected to be smaller. This is supported by the other tests of our study, where losses of these compounds were not detected (e.g. storage stability or sampling efficiency).
3.5 Recoveries from the ozone scrubbers
Figure 7 depicts an example of average amount fractions of the compounds, without (Fig. 7a) and with (Fig. 7b) the ozone scrubber, with (shaded) and without (unshaded) ozone. Much lower amount fractions were detected especially for myrcene, terpinolene and β-caryophyllene without O3 scrubbers when 40 nmol mol−1 of O3 was generated. This was expected since these BVOCs are most reactive with O3.
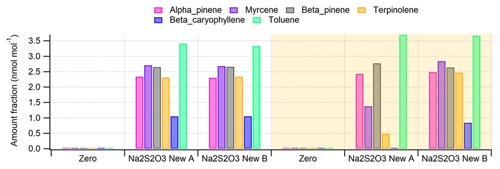
Figure 7Example of amount fractions of studied compounds at each step of the experiment without (a) and with (b) the sodium thiosulfate ozone scrubber. Shaded areas represent periods where ozone was generated with an amount fraction of ∼ 40 nmol mol−1.
The relative differences in samples with and without O3 scrubbers calculated by Eq. (5) are presented for the tests without O3 in Table 5. Generally, new scrubbers were suitable for most of the BVOCs with a relative difference below 5 %, with some exceptions such as for β-farnesene, Na2S2O3 for linalool, and heated SS for linalool and bornyl acetate (Table 5). However, MnO2 scrubbers suffered from the losses of some monoterpenes, all sesquiterpenes and all oxygenated BVOCs, while the recoveries of aromatic hydrocarbons were good. MnO2 scrubbers have been commonly used in monoterpene measurements (e.g. Hakola et al., 2003, 2009), but in earlier tests they have also been found to be unsuitable for sesquiterpenes (Calogirou et al., 1996; Pollmann et al., 2005). In their studies Pollmann et al. (2005) recommended NO titration or sodium-thiosulfate-impregnated filters as suitable O3 removal methods.
Table 5Relative difference between the samples taken with and without new and aged O3 scrubbers for BVOCs and aromatic hydrocarbons and the flow through the scrubber during sampling.
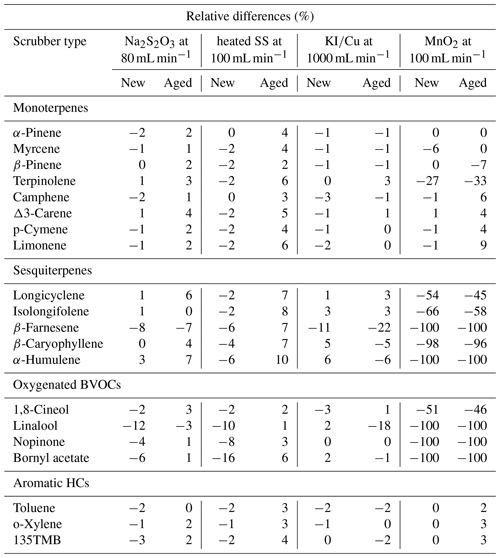
All O3 scrubbers have limited ozone removal capacity, and they must be changed and checked regularly depending on the season, the use and the ozone amount fractions. Since the O3 removal efficiencies and BVOC recoveries are highly dependent on the flows used and sizes of the scrubbers (Hellén et al., 2012b), the results here are valid only for the scrubber types and flows presented in Tables 2 and 5. For SS scrubbers, heating is also essential for both ozone removal and BVOC recovery.
3.6 Recoveries of terpenes from particle filters
The relative differences between the quantities measured without the particle filter and the quantities measured with the filter were calculated (Eq. 6). The results are depicted in Fig. 8.
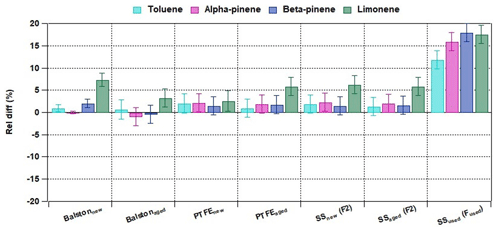
Figure 8Relative difference between the measured quantities without and with the particle filters for terpenes and toluene. Error bars represent 1σ.
Based on the results, the application of a particle filter can affect the measured quantities of monoterpenes, mainly limonene, in the range of ±6 %. All filters types affect limonene more (< +7 %) than α-pinene and β-pinene (±2 %). The higher reactivity of limonene may explain this (Hellén et al., 2018). In addition, it is evident that a filter that has been used in a field campaign for a longer time (SSused (Fused)) compared to a filter that has been charged shortly indoors (SSaged (F2)) can lead to higher differences. Thus, attention should be paid to the types of filters used for ambient measurements as well as to the maximum duration of use.
3.7 Intercomparison of the sorbent tubes
For Tenax TA tubes, the relative standard deviations between the four replicate samples from each set-up (15 or 30 min samples for FMI and IMT) varied in the range of 0.4 %–1.4 %, 0.6 %–2.6 % and 0.4 %–4.6 % for α-pinene, β-pinene and toluene, respectively. The relative difference for α-pinene between measurements conducted for 15 min and the ones conducted for 30 min was 6.8 % and 6.1 % for FMI and IMT results, respectively. These deviations are at the same level as uncertainties (6.8 %, k=2). For β-pinene IMT, 15 and 30 min results were also in agreement (relative difference 5.1 % and uncertainty 6.2 %). The exception was β-pinene for FMI. The 30 min measurements of this compound led to an amount fraction out of the calibration range, which in turn led to a higher relative difference (26 %) between the 15 and 30 min samples of FMI.
For Carbopack B tubes the relative standard deviation for the four replicate samples from each set-up was higher than for the Tenax TA tubes, being 2 %–7 %, 10 %–28 % and 1 %–9 % for α-pinene, β-pinene and toluene, respectively. Additionally, the deviation between 15 and 30 min samples was high for FMI samples, especially for β-pinene (Fig. 9). The comparison between the Tenax TA and Carbopack B tubes showed increased amount fractions of α-pinene and decreased amount fractions of β-pinene in the Carbopack B tubes. In addition to that, both laboratories identified additional compounds (e.g. camphene and p-cymene) in the chromatograms obtained with the Carbopack B tubes. In the Tenax TA tubes no additional terpene peaks were detected. This indication enhances the conclusion that in the Carbopack B tubes, losses of β-pinene can be attributed to the generation of other terpenes due to isomerization. Moreover, toluene showed less obvious differences between the two types of tubes.
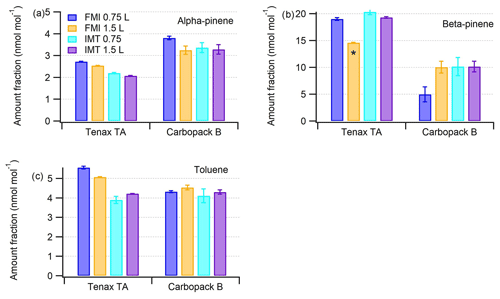
Figure 9Amount fractions (in nmol mol−1) of (a) α-pinene, (b) β-pinene and (c) toluene in Tenax TA and Carbopack B tubes sampled for volumes of 0.75 and 1.5 L and quantified by FMI and IMT Nord Europe laboratories. Error bars represent the average expanded uncertainty (k=2; see Sect. S1) of the four measurements. The asterisk (*) indicates that β-pinene amount fractions were out of the calibration range.
When results of FMI and IMT were compared taking into account uncertainties, the results for α-pinene with both sorbents (except 15 min loading with Carbopack B) and for β-pinene with Tenax TA (except 30 min loading, Fig. 9) were in agreement. More specifically for α-pinene the difference between laboratories was 23 % for Tenax TA for both sampling loadings, while for Carbopack B the relative differences were higher for the 15 min loading (13 %) compared to 30 min loading (1 %). For β-pinene the differences with Tenax TA were 6 % and 24 % for 15 and 30 min loading, respectively. The high difference for the 30 min sample was due to exceedance of the FMI calibration range. For β-pinene in Carbopack B the respective differences became 51 % and 1 %. Finally for toluene loaded in Tenax TA the differences between the two laboratories were 43 % and 20 % for 15 and 30 min sampling loading, respectively, while for Carbopack B both were on the order of 5 %. The IMT results regarding toluene are reproducible between Carbopack B and Tenax TA. FMI had problems in the field blank measurements for Tenax TA tubes, and the high toluene readings could have originated from the blank accumulated during the storage and transport, which was not properly characterized. For other studied compounds no contamination was observed during transport.
Some differences between IMT and FMI were higher (23 % for α-pinene in Tenax TA) than the calculated uncertainty (7 % for both laboratories, Table 1, Sect. S2). A possible reason for this is the different calibration methods and their associated uncertainties: FMI calibrated their TD-GC-MS with methanol solutions, while IMT used a traceable NPL gas standard to calibrate their TD-GC-FID. Comparisons of liquid methanol standard and NPL gas standard in Sect. 3.3 indicated that this can cause up to a 12 % difference for α-pinene results. However, to a lesser extent, transportation through regular posting without cooling may have also had impact on the compounds sampled in the FMI tubes.
Sorbent tube tests showed that Tenax TA in stainless-steel (SS)/SilcoNert 1000 tubes was more suitable for the measurements of BVOCs than Carbopack B tubes, for which significant losses of several studied BVOCs were detected. At the same time, when significant losses of several BVOCs were detected in Carbopack B tubes, the amount fractions of some terpenes (e.g. camphene) increased. This indicates that some terpenes can be isomerized forming other terpenes in the Carbopack B tubes. As shown by the losses of sesquiterpenes, oxygenated BVOCs and some monoterpenes during storage, in comparisons with online sampling and in laboratory intercomparisons, Carbopack B is an unsuitable sorbent for the studied BVOCs.
Based on the earlier literature and results from this study, compounds were found to be stable in Tenax TA tubes for at least 1 month at −20 and at +20 ∘C. Breakthrough tests indicated that α- and β-pinene clearly had lower breakthrough volumes in Tenax TA (4–7 and 8–26 L, respectively) than other terpenes (> 160 L) and the breakthrough volumes in Carbopack B tubes were very high (> 160 L) for all studied BVOCs. SilcoNert 1000 and glass were shown to be the best tube materials, but SS tubes also showed acceptable results due to the low surface area of the tubes when filled with sorbents. Considering the cost of SilcoNert 1000 tubes and fragility of glass tubes, SS tube may be the most convenient choice. Both O3 scrubbers and particle filters may impact the measured amount fractions of BVOCs. While , Na2S2O3 and heated SS scrubbers were found to be suitable for measurements of studied BVOCs, MnO2 scrubbers suffered losses of terpinolene, all studied sesquiterpenes and oxygenated BVOCs. Optimizing the sizes of the scrubbers and sampling flows used is highly important for achieving good removal of O3 and acceptable recoveries of BVOCs. All tested particle filters were shown to have an effect more on limonene than on α-pinene and β-pinene. The results also indicated that a filter that has been used in a field campaign for a longer time can lead to higher differences compared to new filters or a filter that has been recently charged indoors.
Tube sampling with Tenax TA and online sampling were in good agreement for most of the compounds. However, some losses of sesquiterpenes and linalool were detected in the online mode compared to sorbent tube sampling. Sorbent tube calibration with methanol solutions of terpenes was within ±10 % when compared to the NPL gas standard for α- and β-pinene and 1,8-cineol, but for limonene 15 % to 20 % lower-than-expected amounts were found when calibrated with the methanol solutions of terpenes.
The study also included atmospheric oxidation products of monoterpenes (nopinone, 4AMCH) and oxygenated monoterpenes (1,8-cineol, linalool). The results showed that Tenax TA sorbent tubes can also be used for studying them.
The laboratory intercomparison showed that in general, the measured values by the two laboratories were in good agreement for terpenes measured with Tenax TA tubes. However, the comparison between Tenax TA and Carbopack B tubes showed increased amount fractions of α-pinene and decreased amount fractions of β-pinene in Carbopack B tubes. Furthermore, artefacts of additional compounds were observed in the chromatograms of Carbopack B tubes. This indication supports the conclusion that in Carbopack B tubes losses of β-pinene can be attributed to the generation of other terpenes due to isomerization. Moreover, toluene showed less obvious differences between the two types of tubes, which was expected based on earlier studies.
Even though online measurements of VOCs are becoming more common, the use of sorbent tubes is expected to continue due to greater spatial coverage and because no infrastructure is needed for sampling. Our findings showing that Tenax TA tubes are suitable as a sampling method of the offline measurement of most of the BVOCs under study confirm that sorbent tubes are still among the most robust methods for a wide range of speciated terpenes and other BVOCs.
For both offline and online measurements there is still a need for stable SI-traceable terpene standards covering many terpenes at atmospheric amount fraction levels to overcome the matrix issue (methanol solution vs. gas standard) – e.g. only four terpenes were contained in the gaseous SI-traceable standard used for this work.
Data for all figures will be available later from the Zenodo repository at https://doi.org/10.5281/zenodo.10371362 (Hellén et al., 2023).
The supplement related to this article is available online at: https://doi.org/10.5194/amt-17-315-2024-supplement.
HH designed the measurements, performed the data analysis and led the writing of the manuscript. TT, SS and ES conducted the measurements and commented on the manuscript. TS designed the measurements, performed data analysis and wrote the manuscript. MIG supervised the study, helped in designing the measurement campaign, provided the calibration and data analysis of the ReGaS2 generation system, and commented on the manuscript.
The contact author has declared that none of the authors has any competing interests.
Publisher’s note: Copernicus Publications remains neutral with regard to jurisdictional claims made in the text, published maps, institutional affiliations, or any other geographical representation in this paper. While Copernicus Publications makes every effort to include appropriate place names, the final responsibility lies with the authors.
We thank Albert Silvi (FMI) and Aku Helin (FMI) for conducting comparison tests with different tube materials, the ACTRIS CiGas unit at IMT Nord Europe including Thierry Léonardis for conducting the intercomparison and for his technical support, and Laurence Depelchin for performing the particulate filter experiments for terpenes. Anja Claude and Stefan Reiman are thanked for providing sodium-thiosulfate-impregnated filters and MnO2 nets as O3 scrubbers.
This research has been supported by the European Metrology Programme for Innovation and Research (EMPIR) (MetClimVOC, project no. 19ENV06) co-financed by the participating states and by the European Union's Horizon 2020 research and innovation programme.
This paper was edited by Pierre Herckes and reviewed by four anonymous referees.
Acosta Navarro, J. C., Smolander, S., Struthers, H., Zorita, E., Ekman, A. M. L., Kaplan, J. O., Guenther, A., Arneth, A., and Riipinen, I.: Global emissions of terpenoid VOCs from terrestrial vegetation in the last millennium, J. Geophys. Res.-Atmos., 119, 6867–6885, https://doi.org/10.1002/2013JD021238, 2014.
Ahn, J.-H., Kim, K.-H., Szulejko, J. E., Kwon, E. E., and Deep, A.: Characterization of quality assurance properties of biogenic volatile organic compounds with an emphasis on the breakthrough behavior, recovery, and temporal stability, Microchem. J., 125, 142–150, https://doi.org/10.1016/j.microc.2015.11.016, 2016.
Arnts, R. R.: Evaluation of adsorbent sampling tube materials and Tenax-TA for analysis of volatile biogenic organic compounds, Atmos. Environ., 44, 12579–1284, https://doi.org/10.1016/j.atmosenv.2010.01.004, 2010.
Borbon, A., Dominuttu, P., Panopoulou, A., Gros, V., Sauvage, S., Farhat, M., Afif, C., Elguindi, N., Fornaro, A., Granier, C., Hopkins, J. R., Liakadou, E., Nogueira, T., Correa dos Santos, T., Salameh, T., Armangaud, A., Piga, D., and Perrussel, O.: Ubiquity of Anthropogenic Terpenoids in Cities Worldwide: Emission Ratios, Emission Quantification and Implications for Urban Atmospheric Chemistry, J. Geophys. Res.-Atmos., 128, e2022JD037566, https://doi.org/10.1029/2022JD037566, 2023.
Bouvier-Brown, N. C., Goldstein, A. H., Gilman, J. B., Kuster, W. C., and de Gouw, J. A.: In-situ ambient quantification of monoterpenes, sesquiterpenes, and related oxygenated compounds during BEARPEX 2007: implications for gas- and particle-phase chemistry, Atmos. Chem. Phys., 9, 5505–5518, https://doi.org/10.5194/acp-9-5505-2009, 2009.
Calogirou, A., Larsen, B. R., Brussol, C., Duane, M., and Kotzias, D.: Decomposition of terpenes by ozone during sampling on Tenax, Anal. Chem., 68, 1499–1506, https://doi.org/10.1021/ac950803i, 1996.
CEN: EN 14662-1, Standard method for measurement of benzene concentrations. Part 1: Pumped sampling followed by thermal desorption and gas chromatography, European Committee fur Standardization, Brussels, 2005.
Cho, K. S., Lim, Y.-R., Lee, K., Lee, J., Lee, J. H., and Lee, I.-S.: Terpenes from forests and human health, Toxicological Research, 33, 97–106, https://doi.org/10.5487/TR.2017.33.2.097, 2017.
Coggon, M. M., Gkatzelis, G. I., McDonald, B. C., Gilman, J. B., Schwantes, R., Abuhassan, N., Aiken, K. C., Arend, M., Berkoff, T., Brown, S., Campos, T., Dickerson, R. R., Gronoff, G., Hurley, J., Isaacman-VanWertz, G., Koss, A. R., Li, M., McKeen, S. A., Moshary, F., Peischl, J., Pospisilova, V., Ren, X., Wilson, A., Wu, Y., Trainer, M., and Warneke, C.: The human forest: Volatile chemical products enhance urban ozone, P. Natl. Acad. Sci. USA, 118, e2026653118, https://doi.org/10.1073/pnas.2026653118, 2021.
Debevec, C., Sauvage, S., Gros, V., Salameh, T., Sciare, J., Dulac, F., and Locoge, N.: Seasonal variation and origins of volatile organic compounds observed during 2 years at a western Mediterranean remote background site (Ersa, Cape Corsica), Atmos. Chem. Phys., 21, 1449–1484, https://doi.org/10.5194/acp-21-1449-2021, 2021.
Demichelis, A., Heikens, D., and Baldan, A.: Feasibility of generating standard gas mixtures of monoterpenes at trace levels, Poster presentation in GAS2009 conference, NMI Van Swinden Laboratorium (VSL), 11–13 February 2009, Rotterdam, the Netherlands, 2009.
Fick, J., Pommer, L., Andersson, B., and Nilsson, C.: Ozone removal in the sampling of parts per billion levels of Terpenoid compounds: an evaluation of different scrubber materials, Environ. Sci. Technol., 35, 1458–1462, https://doi.org/10.1021/es0001456, 2001.
Gallego, E., Roca, F. J., Perales, J. F., and Guardino, X.: Comparative study of the adsorption performance of a multi-sorbent bed (Carbotrap, Carbopack X, Carboxen 569) and a Tenax TA adsorbent tube for the analysis of volatile organic compounds (VOCs), Talanta, 81, 916–924, https://doi.org/10.1016/j.talanta.2010.01.037, 2010.
Guenther, A. B., Jiang, X., Heald, C. L., Sakulyanontvittaya, T., Duhl, T., Emmons, L. K., and Wang, X.: The Model of Emissions of Gases and Aerosols from Nature version 2.1 (MEGAN2.1): an extended and updated framework for modeling biogenic emissions, Geosci. Model Dev., 5, 1471–1492, https://doi.org/10.5194/gmd-5-1471-2012, 2012.
Hakola, H., Tarvainen, V., Laurila, T., Hiltunen, V., Hellén, H., and Keronen, P.: Seasonal variation of VOC concentrations above a boreal coniferous forest, Atmos. Environ., 37, 1623–1634, https://doi.org/10.1016/S1352-2310(03)00014-1, 2003.
Hakola, H., Tarvainen, V., Bäck, J., Ranta, H., Bonn, B., Rinne, J., and Kulmala, M.: Seasonal variation of mono- and sesquiterpene emission rates of Scots pine, Biogeosciences, 3, 93–101, https://doi.org/10.5194/bg-3-93-2006, 2006.
Hakola, H., Hellén, H., Tarvainen, V., Bäck, J., Patokoski, J., and Rinne, J.: Annual variations of atmospheric VOC concentrations in a boreal forest, Boreal Environ. Res., 14, 722–730, 2009.
Hakola, H., Hellén, H., Hemmilä, M., Rinne, J., and Kulmala, M.: In situ measurements of volatile organic compounds in a boreal forest, Atmos. Chem. Phys., 12, 11665–11678, https://doi.org/10.5194/acp-12-11665-2012, 2012.
Hakola, H., Tarvainen, V., Praplan, A. P., Jaars, K., Hemmilä, M., Kulmala, M., Bäck, J., and Hellén, H.: Terpenoid and carbonyl emissions from Norway spruce in Finland during the growing season, Atmos. Chem. Phys., 17, 3357–3370, https://doi.org/10.5194/acp-17-3357-2017, 2017.
Helin, A., Hakola, H., and Hellén, H.: Optimisation of a thermal desorption–gas chromatography–mass spectrometry method for the analysis of monoterpenes, sesquiterpenes and diterpenes, Atmos. Meas. Tech., 13, 3543–3560, https://doi.org/10.5194/amt-13-3543-2020, 2020.
Hellén, H., Hakola, H., Laurila, T., Hiltunen, V., and Koskentalo, T.: Aromatic hydrocarbon and methyl tert-butyl ether measurements in ambient air of Helsinki (Finland) using diffusive samplers, Sci. Total Environ., 298, 55–64, https://doi.org/10.1016/S0048-9697(02)00168-7, 2002.
Hellén, H., Tykkä, T., and Hakola, H.: Importance of isoprene and monoterpenes in urban air in Northern Europe, Atmos. Environ., 59, 59–66, https://doi.org/10.1016/j.atmosenv.2012.04.049, 2012a.
Hellén, H., Kuronen, P., and Hakola, H.: Heated stainless steel tube for ozone removal in the ambient air measurements of mono-and sesquiterpenes, Atmos. Environ., 57, 35–40, https://doi.org/10.1016/j.atmosenv.2012.04.019, 2012b.
Hellén, H., Praplan, A. P., Tykkä, T., Ylivinkka, I., Vakkari, V., Bäck, J., Petäjä, T., Kulmala, M., and Hakola, H.: Long-term measurements of volatile organic compounds highlight the importance of sesquiterpenes for the atmospheric chemistry of a boreal forest, Atmos. Chem. Phys., 18, 13839–13863, https://doi.org/10.5194/acp-18-13839-2018, 2018.
Hellén, H., Schallhart, S., Praplan, A. P., Tykkä, T., Aurela, M., Lohila, A., and Hakola, H.: Sesquiterpenes dominate monoterpenes in northern wetland emissions, Atmos. Chem. Phys., 20, 7021–7034, https://doi.org/10.5194/acp-20-7021-2020, 2020.
Hellén, H., Praplan, A. P., Tykkä, T., Helin, A., Schallhart, S., Schiestl-Aalto, P. P., Bäck, J., and Hakola, H.: Sesquiterpenes and oxygenated sesquiterpenes dominate the VOC (C5–C20) emissions of downy birches, Atmos. Chem. Phys., 21, 8045–8066, https://doi.org/10.5194/acp-21-8045-2021, 2021.
Hellén, H., Tykkä, T., Schallhart, S., Stratigou, E., and Salameh, T.: Data from: Measurements of atmospheric C10-C15 biogenic volatile organic compounds (BVOCs) with sorbent tubes, Version 1.0.0, Zenodo [data set], https://doi.org/10.5281/zenodo.10371362, 2023.
Helmig, D.: Ozone removal techniques in the sampling of atmospheric volatile organic trace gases, Atmos. Environ., 31, 3635–3651, 1997.
Helmig, D., Bocquet, F., Pollmann, J., and Revermann, T.: Analytical techniques for sesquiterpene emission rate studies in vegetation enclosure experiments, Atmos. Environ., 38, 557–572, https://doi.org/10.1016/j.atmosenv.2003.10.012, 2004.
Ho, S. S. H., Chow, J. C., Watson, J. G., Wang, L., Qu, L., Dai, W., Huang, Y., and Cao, J.: Influences of relative humidities and temperatures on the collection of C2–C5 aliphatic hydrocarbons with multi-bed (Tenax TA, Carbograph 1TD, Carboxen 1003) sorbent tube method, Atmos. Environ., 151, 45–51, https://doi.org/10.1016/j.atmosenv.2016.12.007, 2017.
Iqbal, M., Kim, K. H., and Ahn, J. H.; Monoterpenes released from fruit, plant and vegetable systems, Sensors-Basel, 14, 18286–18301, https://doi.org/10.3390/s141018286, 2014.
ISO 6145-10: Gas analysis – Preparation of calibration gas mixtures using dynamic volumetric methods – Part 10: Permeation method, ISO, https://www.iso.org/standard/25916.html (last access: 12 December 2023), 2002.
ISO/DIS 16017-2: Indoor, ambient and workplace air–Sampling and analysis of volatile organic compounds by sorbent tube/thermal desorption/capillary gas chromatography. Part 2: Diffusive sampling, ISO, https://www.iso.org/standard/29195.html (last access: 21 December 2023), 2003.
Jardine, A. B., Jardine, K. J., Fuentes, J. D., Martin, S. T., Martins, G., Durgante, F., Carneiro, V., Higuchi, N., Manzi, A. O., and Chambers, J. Q.: Highly reactive light-dependent monoterpenes in the Amazon, Geophys. Res. Lett., 42, 1576–1583, https://doi.org/10.1002/2014GL062573, 2015.
Karbiwnyk, C. M., Mills, C. S., Helmig, D., and Birks, J. W.: Minimization of water vapor interference in the analysis of non-methane volatile organic compounds by solid adsorbent sampling, J. Chromatogr. A, 958, 219–229, 2002.
Kennedy, E. R., Fischbach, T. J., Song, R., Eller, P. M., and Shulman, S. A.: Summary of the NIOSH Guidelines for Air Sampling and Analytical Method Development and Evaluation, Analyst, 121, 1163–1169, https://doi.org/10.1039/AN9962101163, 1996.
Kim, T., Song, B., Cho, K. S., and Lee, I.-S.: Therapeutic Potential of Volatile Terpenes and Terpenoids from Forests for Inflammatory Diseases, Int. J. Mol. Sci., 21, 2187, https://doi.org/10.3390/ijms21062187, 2020.
Komenda, M., Parusel, E., Wedel, A., and Koppmann, R.: Measurements of biogenic VOC emissions: sampling, analysis and calibration, Atmos. Environ., 35, 2069–2080, https://doi.org/10.1016/S1352-2310(00)00502-1, 2001.
Li, H., Riva, M., Rantala, P., Heikkinen, L., Daellenbach, K., Krechmer, J. E., Flaud, P.-M., Worsnop, D., Kulmala, M., Villenave, E., Perraudin, E., Ehn, M., and Bianchi, F.: Terpenes and their oxidation products in the French Landes forest: insights from Vocus PTR-TOF measurements, Atmos. Chem. Phys., 20, 1941–1959, https://doi.org/10.5194/acp-20-1941-2020, 2020.
Li, H., Canagaratna, M. R., Riva, M., Rantala, P., Zhang, Y., Thomas, S., Heikkinen, L., Flaud, P.-M., Villenave, E., Perraudin, E., Worsnop, D., Kulmala, M., Ehn, M., and Bianchi, F.: Atmospheric organic vapors in two European pine forests measured by a Vocus PTR-TOF: insights into monoterpene and sesquiterpene oxidation processes, Atmos. Chem. Phys., 21, 4123–4147, https://doi.org/10.5194/acp-21-4123-2021, 2021.
Maceira, A., Vallecillos, L., Borrull, F., and Marcé, R. M.: New approach to resolve the humidity problem in VOC determination in outdoor air samples using solid adsorbent tubes followed by TD-GC–MS, Sci. Total Environ., 599–600, 1718–1727, https://doi.org/10.1016/j.scitotenv.2017.05.141, 2017.
Mermet, K., Sauvage, S., Dusanter, S., Salameh, T., Léonardis, T., Flaud, P.-M., Perraudin, É., Villenave, É., and Locoge, N.: Optimization of a gas chromatographic unit for measuring biogenic volatile organic compounds in ambient air, Atmos. Meas. Tech., 12, 6153–6171, https://doi.org/10.5194/amt-12-6153-2019, 2019.
Mermet, K., Perraudin, E., Dusanter, S., Sauvage, S., Léonardis, T., Flaud, P. M., Bsaibes, S., Kammer, J., Michoud, V., Gratien, A., Cirtog, M., Al Ajami, M., Truong, F., Batut, S., Hecquet, C., Doussin, J. F., Schoemaecker, C., Gros, V., Locoge, N., and Villenave, E.: Atmospheric Reactivity of Biogenic Volatile Organic Compounds in a Maritime Pine Forest during the LANDEX Episode 1 Field Campaign, Sci. Total Environ., 756, 144129, https://doi.org/10.1016/j.scitotenv.2020.144129, 2021.
Oh, G. Y., Park, G.-H., Kim, I.-S., Bae, J.-U., Park, H.-Y., Seo, Y.-G., Yang, S.-I., Lee, J.-K., Jeong, S.-H., and Lee W.-J.: Comparison of major Monoterpene Concentrations in the ambient air of South Korea Forests, Journal of Korean Forest Society, 99, 698–705, 2010.
Pascale, C., Guillevic, M., Ackermann, A., Leuenberger, D., and Niederhauser, B.: Two generators to produce SI-traceable reference gas mixtures for reactive compounds at atmospheric levels, Meas. Sci. Technol., 28, 124002, https://doi.org/10.1088/1361-6501/aa870c, 2017.
Peräkylä, O., Vogt, M., Tikkanen, O.-P., Laurila, T., Kajos, M. K., Rantala, P. A., Patokoski, J., Aalto, J., Yli-Juuti, T., Ehn, M., Sipilä, M., Paasonen, P., Rissanen, M., Nieminen, T., Taipale, R., Keronen, P., Lappalainen, H. K., Ruuskanen, T. M., Rinne, J., Kerminen, V.-M., Kulmala, M., Bäck, J., and Petäjä, T.: Monoterpenes' oxidation capacity and rate over a boreal forest: temporal variation and connection to growth of newly formed particles, Boreal Environ. Res., 19, 293–310, 2014.
PerkinElmer Inc: Technical note: packing thermal desorption sample tubes, PerkinElmer Life and Analytical Sciences, USA, 2007.
Petäjä, T., Tabakova, K., Manninen, A., Ezhova, E., O'Connor, E., Moisseev, D., Sinclair, V., Backman, J., Levula, J., Luoma, K., Virkkula, A., Paramonov, M., Räty, M., Äijälä, M., Heikkinen, L., Ehn, M., Sipilä, M., Yli-Juuti, T., Virtanen, A., Ritsche, M., Hickmon, N., Pulik, G., Rosenfeld, D., Worsnop, D., Back, J., Kulmala, M., and Kerminen, V.-M.: Influence of biogenic emissions from boreal forests on aerosol-cloud interactions, Nat. Geosci., 15, 42–47, https://doi.org/10.1038/s41561-021-00876-0, 2022.
Pollmann, J., Ortega, J., and Helmig, D.: Analysis of atmospheric sesquiterpenes: sampling losses and mitigation of ozone Interferences, Environ. Sci. Technol., 39, 9620–9629, https://doi.org/10.1021/es050440w, 2005.
Reimann, S., Wegener, R., Claude, A., and Sauvage S.: Updated Measurement Guideline for NOx and VOCs, ACTRIS, Deliverable 3.17, https://www.actris.eu/sites/default/files/inline-files/WP3_D3.17_M42_0.pdf (last access: 12 December 2023), 2018.
Schallhart, S., Rantala, P., Kajos, M. K., Aalto, J., Mammarella, I., Ruuskanen, T. M., and Kulmala, M.: Temporal variation of VOC fluxes measured with PTR-TOF above a boreal forest, Atmos. Chem. Phys., 18, 815–832, https://doi.org/10.5194/acp-18-815-2018, 2018.
Sheu, R., MArcotte, A., Khare, P., Charan, S., Ditto, J. C., and Gentner, D. R.: Advances in offline approaches for chemically speciated measurements of trace gas-phase organic compounds via adsorbent tubes in an integrated sampling-to-analysis system, J. Chromatogr. A, 1575, 80–90, https://doi.org/10.1016/j.chroma.2018.09.014, 2018.
Sindelarova, K., Granier, C., Bouarar, I., Guenther, A., Tilmes, S., Stavrakou, T., Müller, J.-F., Kuhn, U., Stefani, P., and Knorr, W.: Global data set of biogenic VOC emissions calculated by the MEGAN model over the last 30 years, Atmos. Chem. Phys., 14, 9317–9341, https://doi.org/10.5194/acp-14-9317-2014, 2014.
Song, W., Williams, J., Yassaa, N., Martinez, M., Carnero, J. A. A., Hidalgo, P. J., Bozem, H., and Lelieved, J.: Winter and summer characterization of biogenic enantiomeric monoterpenes and anthropogenic BTEX compounds at a Mediterranean Stone Pine forest site, J. Atmos. Chem., 68, 233–250, https://doi.org/10.1007/s10874-012-9219-4, 2012.
Steinbrecher, R. and Weiß, E.: Standard Operating Procedures (SOPs) for Air Sampling in Stainless Steel Canisters for Non-Methane Hydrocarbons Analysis, World Meteorological Organization, GAW report No. 204, https://library.wmo.int/es/records/item/48749-standard- operating-procedures-sops-for-air-sampling-in-stainless-steel-canisters-for-non-methane-hydrocarbons-analysis (last access: 12 December 2023), 2012.
Steinemann, A.: Volatile emissions from common consumer products, Air Qual. Atmos. Hlth., 8, 273–281, https://doi.org/10.1007/s11869-015-0327-6, 2015.
Sunesson, A.-L., Sundgren, M., Levin, J.-O., Eriksson, K., and Carlson, R.: Evaluation of two adsorbents for diffusive sampling and thermal desorption-gas chromatographic analysis of monoterpenes in air, J. Environ. Monitor., 1, 45–50, https://doi.org/10.1039/A807657J, 1999.
Ullah, M. A. and Kim, K.-H.: Performance test of a sorbent tube sampler with respect to analyte loss in collecting biogenic volatile organic compounds, Anal. Bioanal. Chem., 407, 415–426, https://doi.org/10.1007/s00216-014-8258-x, 2014.
Veenas, C., Ripszam, M., and Haglund, P.: Analysis of volatile organic compounds in indoor environments using thermal desorption with comprehensive two-dimensional gas chromatography and high-resolution time-of-flight mass spectrometry, J. Sep. Sci., 43, 1489–1498, https://doi.org/10.1002/jssc.201901103, 2020.
Volden, J., Thomassen, Y., Greibrokk, T., Thorud, S., and Molander, P.: Stability of workroom air volatile organic compounds on solid adsorbents for thermal desorption gas chromatography, Anal. Chim. Acta, 530, 263–271, https://doi.org/10.1016/j.aca.2004.09.019, 2005.
Wilkinson, M., White, I. R., Goodacre, R., Nijsen, T., and Fowler, S. J.: Effects of high relative humidity and dry purging on VOCs obtained during breath sampling on common sorbent tubes, J. Breath Res., 14, 046006, https://doi.org/10.1088/1752-7163/ab7e17, 2020.
Yáñez-Serrano, A. M., Nölscher, A. C., Bourtsoukidis, E., Gomes Alves, E., Ganzeveld, L., Bonn, B., Wolff, S., Sa, M., Yamasoe, M., Williams, J., Andreae, M. O., and Kesselmeier, J.: Monoterpene chemical speciation in a tropical rainforest:variation with season, height, and time of dayat the Amazon Tall Tower Observatory (ATTO), Atmos. Chem. Phys., 18, 3403–3418, https://doi.org/10.5194/acp-18-3403-2018, 2018.
Yee, L. D., Isaacman-VanWertz, G., Wernis, R. A., Meng, M., Rivera, V., Kreisberg, N. M., Hering, S. V., Bering, M. S., Glasius, M., Upshur, M. A., Gray Bé, A., Thomson, R. J., Geiger, F. M., Offenberg, J. H., Lewandowski, M., Kourtchev, I., Kalberer, M., de Sá, S., Martin, S. T., Alexander, M. L., Palm, B. B., Hu, W., Campuzano-Jost, P., Day, D. A., Jimenez, J. L., Liu, Y., McKinney, K. A., Artaxo, P., Viegas, J., Manzi, A., Oliveira, M. B., de Souza, R., Machado, L. A. T., Longo, K., and Goldstein, A. H.: Observations of sesquiterpenes and their oxidation products in central Amazonia during the wet and dry seasons, Atmos. Chem. Phys., 18, 10433–10457, https://doi.org/10.5194/acp-18-10433-2018, 2018.
Zannoni, N., Gros, V., Lanza, M., Sarda, R., Bonsang, B., Kalogridis, C., Preunkert, S., Legrand, M., Jambert, C., Boissard, C., and Lathiere, J.: OH reactivity and concentrations of biogenic volatile organic compounds in a Mediterranean forest of downy oak trees, Atmos. Chem. Phys., 16, 1619–1636, https://doi.org/10.5194/acp-16-1619-2016, 2016.