the Creative Commons Attribution 4.0 License.
the Creative Commons Attribution 4.0 License.
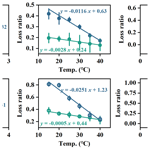
Calibrating adsorptive and reactive losses of monoterpenes and sesquiterpenes in dynamic chambers using deuterated surrogates
Jianqiang Zeng
Haofan Ran
Weihua Pang
Hao Guo
Zhaobin Mu
Wei Song
Xinming Wang
Accurately measuring the emissions of monoterpenes (MTs) and sesquiterpenes (SQTs) using dynamic chambers requires careful consideration of their adsorptive and reactive losses, which are often overlooked and difficult to assess in situ. This study evaluated the effectiveness of deuterated surrogates, α-pinene-d3 and β-caryophyllene-d2, in tracing these losses in a dynamic chamber system. Using standard gas mixtures of 10 MTs and 10 SQTs, we characterized adsorptive losses across varying concentrations, temperatures, and humidity levels, as well as reactive losses with ozone. Results indicated that adsorptive losses were significantly influenced by concentration and temperature, with species-specific variations particularly under low concentrations and low temperatures, while relative humidity had negligible impact. Reactive losses with ozone exhibited substantial species-specific variability. Key MTs (α-pinene, β-pinene, 3-carene, limonene, and 1,8-cineole) and SQTs (β-caryophyllene and α-humulene) demonstrated consistent adsorptive and reactive behaviors with their respective deuterated surrogates α-pinene-d3 and β-caryophyllene-d2, suggesting that these surrogates are effective for correcting losses with in situ emission measurements using dynamic chambers. However, due to varied adsorptive and reactive losses, additional deuterated MTs and SQTs are recommended, particularly selected according to their O3 reactivities, to cover a broader range of MTs and SQTs for loss correction. A strong correlation between adsorptive capacity and ozone reactivity was observed, underscoring the need to carefully address losses of highly reactive MTs and SQTs during emission measurements. This study also emphasizes that ozone-free circulating air should be used for accurately measuring emissions of highly reactive SQTs, such as β-caryophyllene and α-humulene, especially when loss correction methods are unavailable.
- Article
(2620 KB) - Full-text XML
-
Supplement
(1644 KB) - BibTeX
- EndNote
Monoterpenes (MTs) and sesquiterpenes (SQTs) emitted from terrestrial vegetation account for about 18 % of global biogenic volatile organic compound (BVOC) emissions, with even higher contributions in certain ecosystems (Guenther et al., 2012). These reactive organic gases can rapidly react with atmospheric oxidants such as ozone (O3), hydroxyl radicals (OH), and nitrate radicals (NO3) (Di Carlo et al., 2004; Jardine et al., 2011; Edwards et al., 2017; He et al., 2021), influencing atmospheric oxidation capacity and leading to the formation of organic aerosols, which, as significant components of fine particles, can scatter solar radiation and act as cloud condensation nuclei (Yli-Juuti et al., 2021), directly and indirectly affecting air quality and climate (Peñuelas and Staudt, 2010; Unger, 2014; Scott et al., 2017).
Despite their importance, current BVOC emission models carry large uncertainties, largely due to inaccurate emission factors (Guenther et al., 2006, 2012). Common BVOC emission measurement techniques, such as leaf cuvettes and dynamic branch chambers (Niinemets et al., 2011; Šimpraga et al., 2019), are prone to adsorptive and reactive losses, particularly for the less volatile MTs and SQTs (Ortega et al., 2008). Their adsorptive losses, which can vary with conditions like temperature and concentration, may lead to significant underestimation of emission rates (Niinemets et al., 2011; Zeng et al., 2022a). For example, Helmig et al. (2004) reported that, after a 5 h equilibrium, only 80 % of SQTs and 60 % of oxygenated SQTs were recovered in a leaf cuvette, requiring 5–10 h to purge to background levels. Additionally, MTs and SQTs have short atmospheric lifetimes (from minutes to hours) due to their high reactivity with OH and O3 (Atkinson and Arey, 2003). Field studies have shown significant in-canopy losses of SQTs (up to 61 %) due to O3 in the Amazonian rainforest (Jardine et al., 2011). These findings highlight the risk of underestimating the emissions of reactive terpenes using existing methods, especially in dynamic chambers where adsorptive and reactive losses remain a challenge (Niinemets et al., 2011).
Dynamic chambers, commonly made from chemically inert materials like Teflon or Tedlar, minimize but do not eliminate these losses (Niinemets et al., 2011; Materic et al., 2015). Previous studies have reported that adsorptive losses of MTs and SQTs in such chambers can exceed 30 %, even with residence times of the chamber under 1 min (Zeng et al., 2022a). Field studies also revealed that adsorptive losses in Tedlar bags reached 20 %–30 % for MTs and ranged 10 %–80 % for SQTs (Ortega et al., 2008). Temperature plays a critical role, with more substantial adsorptive losses at lower temperatures (Schaub et al., 2010). The adsorbed compounds may re-emit at higher temperatures, leading to potential overestimation (Ruuskanen et al., 2005; Schaub et al., 2010). Meanwhile, the presence of O3 complicates measurements, as it both influences BVOC emissions from plants and reacts with MTs and SQTs (Feng et al., 2019; Zeng et al., 2022b). Some studies have used O3-free circulating air to mitigate the reactive losses (Helmig et al., 2007; Ortega et al., 2008; Hellén et al., 2021), but this may neglect the impact of O3 on BVOC emissions. In contrast, the use of ambient air can reflect real-world conditions (Kuhn et al., 2002; Bourtsoukidis et al., 2012; Zeng et al., 2022b), but the reactive loss exists. Reducing residence time by increasing the flow rate of circulating air can lower the reactive losses (Zeng et al., 2022a), but it may still not be suitable for highly reactive MTs and SQTs (Pollmann et al., 2005; Niinemets et al., 2011).
Addressing these issues is crucial for improving BVOC emission measurements. While previous laboratory studies have evaluated adsorptive losses in empty chambers (Kolari et al., 2012; Hohaus et al., 2016; Helin et al., 2020; Ghirardo et al., 2020; Zeng et al., 2022a; He et al., 2024) and proposed correction methods for simplified chamber systems (Ghirardo et al., 2020; Zeng et al., 2022a; He et al., 2024), variations in chamber design, plant species, and environmental conditions introduce additional complexities for field measurements. To address these challenges, internal surrogates are recommended for evaluating and correcting such losses in dynamic chambers (Ortega et al., 2008; Zeng et al., 2022a). While internal surrogates like aromatic compounds have been used to assess adsorptive losses, their suitability as surrogates for MTs and SQTs remains debated (Helmig et al., 2007; Ortega et al., 2008). More importantly, the reactivity of these compounds with O3 in dynamic chambers has not been thoroughly evaluated (Pollmann et al., 2005; Helin et al., 2020; Zeng et al., 2022a), and this reactive loss for individual MT and SQT species cannot be assessed by using surrogates like aromatic compounds that have quite different reactivities with O3.
This study investigates the potential of using deuterated α-pinene-d3 and β-caryophyllene-d2 to quantify the adsorptive and reactive losses of individual MTs and SQTs in dynamic chambers, taking advantage of the almost identical adsorption and reaction behaviors of these deuterated compounds as their counterpart MTs and SQTs. We evaluated these losses of α-pinene-d3 and β-caryophyllene-d2, along with target MTs and SQTs, under varying concentration, temperature, and humidity, and we analyzed the gas-phase reactive losses with O3 at different concentrations.
2.1 Lab evaluation
A cylindrical dynamic chamber, which is made of poly(methyl methacrylate) with the inner surface coated with a fluorinated ethylene propylene (FEP) (Teflon) film (FEP 100, type 200A, DuPont, Witton, USA), was used for lab evaluation. It has a diameter of 25 cm and a height of 28 cm, yielding a volume of 13.7 L. A detailed information about the design and initial characterization of this dynamic chamber was described in our previous study (Zeng et al., 2022a). Here, we used standard gas mixtures of α-pinene-d3, β-caryophyllene-d2, 10 species of MTs, and 10 species of SQTs to simulate their emissions from enclosed leaves in the chamber. Detailed description of the preparation of the standard gas mixtures can be found in Zeng et al. (2022a), and the information on standards is provided in Table S1 in the Supplement. As shown in Fig. S1, when the chamber reached a steady state, offline samples were collected simultaneously from the inlet (C0) and outlet (C1) by commercial adsorbent cartridges (Tenax TA/Carbograph 5TD, Markes International Ltd., Bridgend, UK) connected to a portable dual-channel sampler (ZC-QL, Zhejiang Hengda Instrumentation Ltd., Zhejiang, China) at a rate of 200 mL min−1 for 5 min. Therefore, the recovery was expressed as in percentage (Fig. S1). More detail descriptions of the experiment are provided in Sect. S1 in the Supplement.
The effect of concentration on adsorptive losses was evaluated under four concentration levels, with total mixing ratios of MTs and SQTs of 0.23 and 0.26, 0.37 and 0.47, 0.68 and 0.94, and 2.26 and 3.16 ppb, respectively. These experiments were conducted in O3-free air at a temperature of approximately 25 °C and a relative humidity of about 50 %. The temperature effect was tested at two MTs and SQTs concentration levels: a low concentration of 0.22 and 0.28 ppb and a high concentration of 2.26 and 3.16 ppb. In these experiments, the relative humidity was about 50 % but varied slightly with temperature, while the circulating air was O3-free. For the low-concentration experiments, the temperature ranged from 15 to 40 °C in 5 °C increments, whereas the high-concentration experiments started at 10 °C and ended at 45 °C, also in 5 °C increments. The humidity effect was tested under both low and high concentration levels, similar to the temperature experiments. These humidity experiments were conducted at low (5 %), mid (50 %), and high (95 %) relative humidity (RH) levels, with a constant temperature of about 25 °C and O3-free circulating air. Additionally, the ozone effect was assessed at six ozone mixing ratios of 0, 30, 45, 60, 100, and 200 ppb, with temperature of about 25 °C and RH of approximately 50 %. For each experimental setting, three replicates were performed, and the recovery for a single compound is shown as the mean of these replicates, with error bars representing the standard deviations.
2.2 Lab analysis
Cartridge samples were analyzed by an automatic thermal desorption system (TD-100, Markes International Ltd., Bridgend, UK) coupled to a model 7890 gas chromatograph (GC) with a model 5975 mass-selective detector (MSD) (Agilent Technologies, Inc., CA, USA). The adsorbent cartridges were thermally desorbed by the TD-100 at 280 °C for 10 min, and then the desorbed analytes were transferred by pure helium into a cryogenic trap (U-T11PGC-2S, Markes International Ltd., Bridgend, UK) at −10 °C. Then the trap was rapidly heated to transfer the analytes to the GC/MSD system with an HP-5MS capillary column (30 m × 0.25 mm × 0.25 µm, Agilent Technologies, Inc., CA, USA). The GC oven temperature was programmed to be initially at 35 °C (held for 3 min), increased to 100 °C at 5 °C min−1 and held for 1 min, then to 120 °C at 10 °C min−1 and held for 12 min, and then to 260 °C with a final hold time of 2 min. The MSD was operated simultaneously under scan mode and selected ion monitoring mode with electron impacting ionization at 70 eV. Target compounds were identified by comparing their retention times with authentic standards (Table S1) and were quantified with the standard calibration curves. More detailed descriptions of the identification and quantification can be found in our previous studies (Zeng et al., 2022b, 2023, 2024) and in Sect. S2. Method detection limits (MDLs) were determined by seven parallel analyses of the lowest concentration of calibration standards. Based on a sample volume of 1 L, the MDLs varied from 8 to 92 ng m−3 for MTs and from 2 to 92 ng m−3 for SQTs, and the measurement precision ranged from 2.3 % to 4.8 % for MTs and from 1.1 % to 3.4 % for SQTs (Table S2).
3.1 Concentration effect
The adsorptive behaviors of different MTs and SQTs varied significantly across concentration levels (Fig. 1). At low concentrations, recoveries ranged from 59 % for γ-terpinene to 81 % for α-pinene among MTs and from 40 % for α-humulene to 71 % for longicyclene among SQTs (Fig. 1a). In contrast, under high concentration levels, recoveries were more consistent, ranging from 75 % for β-myrcene to 87 % for β-pinene among MTs and from 74 % for β-chamigrene to 84 % for α-longipinene for SQTs (Fig. 1d). Overall, recoveries were higher at elevated concentrations (Figs. S3 and S4), with average recoveries of 68 ± 9 %, 75 ± 7 %, 78 ± 5 %, and 84 ± 2 % for all MTs and 58 ± 8 %, 63 ± 5 %, 71 ± 4 %, and 82 ± 1 % for all SQTs across four concentration levels (Fig. S5).
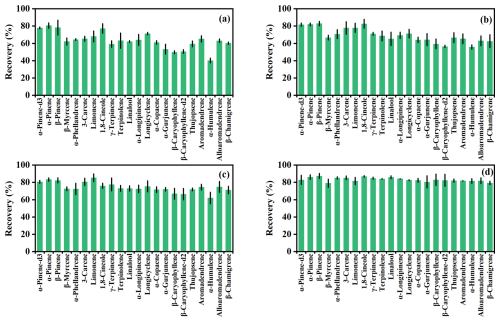
Figure 1Recoveries of MT and SQT species under four different concentration levels. Total MTs and SQTs mixing ratios are 0.23 and 0.26 ppb (a), 0.37 and 0.47 ppb (b), 0.68 and 0.94 ppb (c), and 2.26 and 3.16 ppb (d), respectively.
Despite using chemically inert materials in chamber construction, significant adsorptive losses of MTs and SQTs persist (Ortega et al., 2008; Niinemets et al., 2011; Kolari et al., 2012; Zeng et al., 2022a). Our findings corroborate previous studies (Ortega et al., 2008; Niinemets et al., 2011), demonstrating that low concentrations lead to higher relative losses (Fig. 1). In steady-state conditions, the amount of adsorbed MTs and SQTs is constant, implying that lower concentration results in a greater proportion of total loss (Figs. S3 and S4). This suggests that low-emitting plants, especially for SQTs, may experience substantial adsorptive losses.
We observed species-specific recovery differences at low concentrations (Fig. 1a), likely due to their physicochemical properties (Niinemets et al., 2004, 2011). Less reactive MTs like α-pinene-d3, α-pinene, β-pinene, and 1,8-cineole exhibited stable recoveries regardless of concentration changes. In contrast, more reactive MTs, such as β-myrcene, α-phellandrene, γ-terpinene, and terpinolene, showed significant recovery variations (Fig. S3). This pattern also applies to SQTs, with highly reactive compounds, like β-caryophyllene, β-caryophyllene-d2, and α-humulene, being more affected than others (Fig. S4). These compound-specific adsorptive losses indicate that α-pinene-d3 and β-caryophyllene-d2 cannot serve as surrogates for all MTs and SQTs, respectively.
3.2 Temperature effect
Overall recoveries of MTs and SQTs were higher at elevated temperatures, with a smaller absolute effect observed at high concentrations compared to low concentrations (Figs. 2 and S6). At the high concentration, mean recoveries increased from 80 ± 4 % to 91 ± 1 % for MTs and from 73 ± 3 % to 89 ± 3 % for SQTs as temperature rose from 10 to 45 °C (Figs. S7 and S8). At the low concentration, temperature influenced recoveries more significantly (Fig. 2), with mean values rising from 70 ± 12 % to 85 ± 4 % for MTs and from 50 ± 21 % to 77 ± 6 % for SQTs as temperature increased from 15 to 40 °C. At low concentration and low temperatures (e.g., 15 °C), the standard deviation of recoveries was greater, such as 12 % for MTs and 21 % for SQTs, indicating variability among species (Fig. 2). However, at low concentration but high temperatures (e.g., 40 °C), recoveries became more consistent, with reduced standard deviation of 4 % for MTs and 6 % for SQTs (Fig. 2).
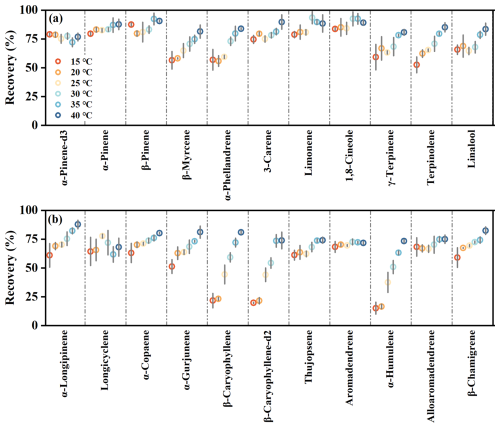
Figure 2Recoveries of different MT (a) and SQT (b) species under different temperatures under the low concentration level.
The temperature effect on recovery was compound-specific. Among MTs, β-myrcene, α-phellandrene, γ-terpinene, terpinolene, and linalool showed greater sensitivity (Fig. 2a), while β-caryophyllene, β-caryophyllene-d2, and α-humulene showed greater sensitivity among SQTs (Fig. 2b). We categorized MTs and SQTs into two groups based on their adsorptive behaviors (Fig. 3). MTs in group 1 included α-pinene-d3, α-pinene, β-pinene, 3-carene, limonene, and 1,8-cineole, while MTs in group 2 consisted of β-myrcene, α-phellandrene, γ-terpinene, terpinolene, and linalool. SQTs in group 1 comprised α-longipinene, longicyclene, α-copaene, α-gurjunene, thujopsene, aromadendrene, alloaromadendrene, and β-chamigrene, whereas SQTs in group 2 included β-caryophyllene, β-caryophyllene-d2, and α-humulene. Under the high concentration, temperature changes minimally affected recoveries, with slopes of 0.37 ± 0.07 % °C−1 for all MTs and 0.43 ± 0.08 % °C−1 for all SQTs (Fig. 3). However, under the low concentration, sensitivity varied significantly between groups, with slopes of 0.27 ± 0.06 % °C−1 for MTs and 0.53 ± 0.05 % °C−1 for SQTs in group 1 much lower than those 1.12 ± 0.12 % °C−1 for MTs and 2.51 ± 0.19 % °C−1 for SQTs in group 2 (Fig. 3).
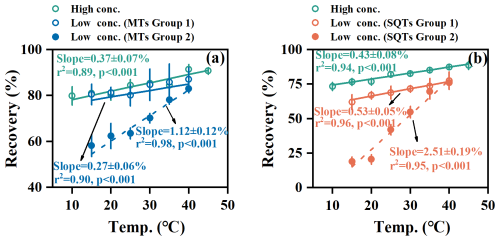
Figure 3Variations of recoveries of MTs and SQTs along with temperature under low and high concentration levels. MTs in group 1 include α-pinene-d3, α-pinene, β-pinene, 3-carene, limonene, and 1,8-cineole, while MTs in group 2 include β-myrcene, α-phellandrene, γ-terpinene, terpinolene, and linalool. α-Longipinene, longicyclene, α-copaene, α-gurjunene, thujopsene, aromadendrene, and alloaromadendrene belong to SQT group 1, while β-caryophyllene, β-caryophyllene-d2, and α-humulene belong to SQT group 2.
Our results demonstrate that adsorptive losses of MTs and SQTs are influenced by both concentration and temperature. Elevated temperatures facilitate evaporation and diffusion, reducing adsorptive losses (Niinemets et al., 2004; Ortega and Helmig, 2008). Field measurements on Picea pungens have similarly shown increased recoveries at high temperatures (Ortega et al., 2008). At low concentrations, the pronounced sensitivity to temperature results in greater recovery differences among species particularly at lower temperatures (Fig. 3), indicating that α-pinene-d3 and β-caryophyllene-d2 may not effectively calibrate adsorptive losses across all MTs and SQTs. Since the inter-group adsorptive behaviors (e.g., MTs in group 1 vs. in group 2) differ significantly but the intra-group adsorptive behaviors for both MTs and SQTs remain consistent (Fig. 3), this suggests that α-pinene-d3 and β-caryophyllene-d2 instead can be used to calibrate the adsorptive losses for MTs in group 1 (α-pinene, β-pinene, 3-carene, limonene, and 1,8-cineole) and SQTs in group 2 (β-caryophyllene and α-humulene), respectively.
3.3 Humidity effect
The impact of humidity on the adsorptive losses of MTs and SQTs was examined at three RH levels: 5 %, 50 %, and 95 %. Overall recoveries were slightly higher at 95 % RH compared to lower RH levels (Fig. 4). For low-concentration experiments, mean recoveries of MTs and SQTs were 72 ± 9 % and 64 ± 20 % at 5 % RH, 72 ± 10 % and 62 ± 13 % at 50 % RH, and 80 ± 6% and 70 ± 15% at 95 % RH, respectively (Fig. 4a), while for high-concentration experiments they were 77 ± 2 % and 76 ± 2 % at 5 % RH, 79 ± 5 % and 82 ± 2% at 50 % RH , and 82 ± 2 % and 82 ± 2 % at 95 %, respectively (Fig. 4b), with recoveries more consistent across RH levels.
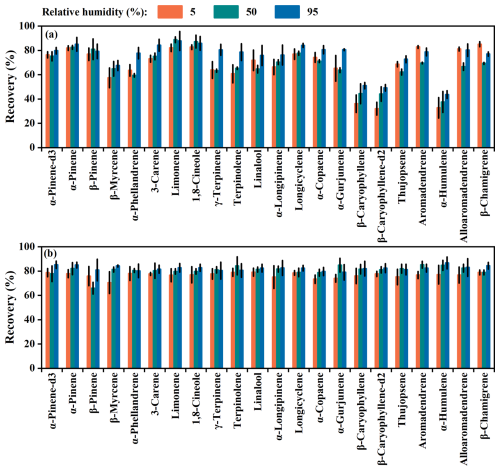
Figure 4Recoveries of different MT and SQT species under different relative humidity levels; (a) low concentration level and (b) high concentration level.
The results suggest that humidity has a minor impact on adsorptive loss, aligning with previous findings (Hohaus et al., 2016; Helin et al., 2020; Zeng et al., 2022a). The relationship between adsorptive losses and humidity is complex, as water molecules can compete with MTs and SQTs for adsorption sites, potentially reducing the adsorption of hydrophobic MTs and SQTs (Kolari et al., 2012; Zeng et al., 2022a). These results indicate that the effect of humidity on the adsorptive loss of MTs and SQTs in dynamic chambers can be considered negligible during field studies.
3.4 Ozone effect
The impact of O3 on the reactive loss of MTs and SQTs was evaluated at six mixing ratio levels: 0, 30, 45, 60, 100, and 200 ppb. Recoveries of α-pinene-d3, α-pinene, β-pinene, 3-carene, and 1,8-cineole remained stable across O3 levels. However, recoveries of β-myrcene, limonene, and γ-terpinene declined slightly with elevated O3 levels, and more pronounced losses were observed for α-phellandrene and terpinolene, with recoveries of 70 ± 3 % and 78 ± 2 % at an O3 mixing ratio of 60 ppb, respectively (Fig. S9). For SQTs, longicyclene, α-copaene, aromadendrene, and alloaromadendrene exhibited relatively stable recoveries, while α-longipinene, α-gurjunene, thujopsene, and β-chamigrene suffered significant losses (Fig. 5). Notably, the recoveries of β-caryophyllene, β-caryophyllene-d2, and α-humulene dropped sharply with increasing O3 levels, with recoveries as low as 41 ± 3 %, 36 ± 6 %, and 30 ± 4 %, respectively, even at a low O3 level of 30 ppb (Fig. 5).
Given ozone's role in reactive losses, some studies have used O3-free circulating air during field measurements to mitigate these effects (Ortega et al., 2008; Helmig et al., 2013). However, it is also critical to understand ozone's role in affecting emissions of MTs and SQTs (Bourtsoukidis et al., 2012; Feng et al., 2019; Zhang et al., 2022). Since the measured emissions may not reflect real-world emissions if O3 is cleaned, some studies have used ambient air as circulating air during field measurements (Kuhn et al., 2002; Bourtsoukidis et al., 2012; Zeng et al., 2022b). The relationship between reactive loss and O3 concentration can be modeled by
where C0 (molecule cm−3) and Ct (molecule cm−3) are the initial and time t concentrations in the chamber, respectively; k (cm3 molecule−1 s−1) is the reaction rate constant; t (s) is the reaction time of the compound in the chamber; and [O3] (molecule cm−3) represents the concentration of O3. In this study, when the Ct reached equilibrium concentration (Cs) with a reaction time of the residence time, Eq. (1) can be rewritten as
where V (L) is the volume of the chamber, and F (L s−1) is the flow rate of circulating air. As a result, through the application of a linear regression between −ln () and [O3], we can derive k values for MTs and SQTs, as illustrated in Fig. S10. However, k values for some less reactive MTs and SQTs cannot be obtained this way and Table 1 presents the k values of some highly reactive species, while literature-reported k values are shown in Table S3. In the present study, the k values of β-myrcene, α-phellandrene, limonene, and terpinolene were 5.93 ± 0.17, 28.15 ± 1.11, 3.06 ± 0.14, and 19.21 ± 1.20 () cm3 molecule−1 s−1, respectively, which are in agreement with those reported in the literature (Table 1). For SQTs, the k values were 20.17 ± 0.98, 14.56 ± 0.15, 115.33 ± 3.83, 129.14 ± 4.42, 8.64 ± 0.16, 147.04 ± 3.87, and 15.14 ± 0.16 () cm3 molecule−1 s−1 for α-longipinene, α-gurjunene, β-caryophyllene, β-caryophyllene-d2, thujopsene, α-humulene, and β-chamigrene, respectively. The k values for the most important β-caryophyllene and α-humulene were consistent with those reported previously (Table 1). The good consistency of k values with the literature proves that the assessment of reactive losses with O3 in the dynamic chamber used here is reliable.
Table 1The measured and literature-reported rate constants (k, cm3 molecule−1 s−1) of MTs and SQTs with ozone.
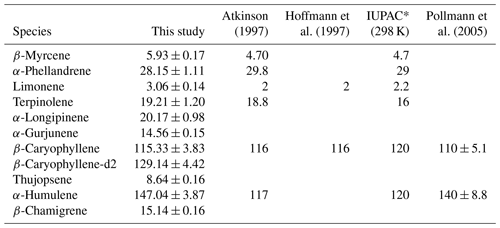
* IUPAC Task Group on Atmospheric Chemical Kinetic Data Evaluation (http://iupac.pole-ether.fr, last access: 28 September 2024).
The compound-specific reactive losses due to their varying reactivity with O3 (Table 1) necessitates their consideration during field measurements, particularly for highly reactive species like β-caryophyllene and α-humulene. Reactive loss caused by O3 can be reduced by shortening the residence time through increasing the flow rate of circulating air. For the chamber in this study, with a flow rate of 9 L min−1 for circulating air (∼ 1.5 min of residence time), the reactive losses of all tested MTs, except for α-phellandrene and terpinolene, were less than 10 %, even at a high O3 mixing ratio of 100 ppb (Fig. S9). This suggests that the effect of O3 on the reactive losses of main MTs (e.g., α-pinene, β-pinene, limonene) can be disregarded during field measurements. For SQTs, although the reactive losses for longicyclene, α-copaene, aromadendrene, and alloaromadendrene were less than 10 % at 100 ppb O3, more than 60 % of the critical species like β-caryophyllene and α-humulene were consumed by O3 even at a low mixing ratio of 30 ppb (Fig. 5). As shown in Fig. S11, to limit the reactive loss of β-caryophyllene within 15 %, the flow rate needs to exceed 42 L min−1, while the O3 mixing ratio should be kept below 30 ppb. Although the detection of instrument is not a problem even under this high flow rate (Zeng et al., 2022a), the requirement for an O3 mixing ratio below 30 ppb poses practical challenges, as this level is already lower than those observed in most background regions worldwide (Lu et al., 2018; Wang et al., 2019). This suggests that using O3-free circulating air may be more suitable when measuring emissions of highly reactive SQTs in the field, as a short-term O3 scavenge during measurements could have negligible impact on the BVOC emissions as demonstrated by Niinemets et al. (2011).
3.5 Implications for field measurements
As discussed above and illustrated in Fig. 6, the effects of concentration, temperature, and O3 on the losses of MTs and SQTs are compound-specific. The adsorptive and reactive losses in group 2 are generally greater than those in group 1, reflecting their different physicochemical properties (Niinemets et al., 2011). Notably, we observed a positive correlation between adsorptive capacity and O3 reactivity (Fig. 7), suggesting that compounds with higher O3 reactivity experience greater temperature- and concentration-driven adsorptive losses. These findings underscore the importance of accounting for both reactive and adsorptive losses in accurately measuring emissions of highly reactive MTs and SQTs.
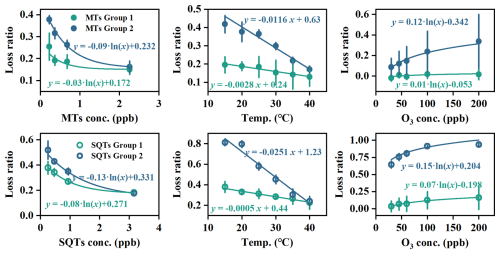
Figure 6The relationships between loss ratio of different MT and SQT groups with concentration, temperature, and ozone mixing ratio.
Furthermore, findings here demonstrate temperature as a crucial factor in influencing the adsorptive losses of terpenes especially SQTs in dynamic chambers. For instance, assuming leaves with a dry mass of 10 g are enclosed in the chamber, the lowest concentration evaluated here, equivalent to emission rates of 0.07 µg g−1 h−1 for MTs and 0.12 µg g−1 h−1 for SQTs, represents a low MT-emitting but high SQT-emitting plant (Duhl et al., 2008; Ortega et al., 2008). Despite being a low MT emitter, at temperatures below 30 °C, the adsorptive losses for MTs in group 1 are only about 20 %, while those in group 2 exceed 30 %. In contrast, adsorptive losses for SQTs in groups 1 and 2 can be greater than 30 % and 45 %, respectively, even for a high SQT-emitting plant. This suggests that in high-latitude regions with relatively cold weather although adsorptive losses of some key MTs, such as those in group 1, may be acceptable, losses can be significant for SQTs, especially the most important β-caryophyllene and α-humulene, thus needing loss corrections during measurements. Moreover, in tropical and subtropical regions characterized by hot weather, high daytime temperatures and strong sunlight can cause temperatures in dynamic chambers to exceed 35 °C, even surpassing 45 °C during heat events (Zeng et al., 2022b; Doughty et al., 2023). Under these conditions, adsorptive losses can be reduced to within 20 % for most MTs and 25 % for most SQTs, regardless of changes in concentration and humidity. This indicates that adsorptive losses of MTs and SQTs may not pose a significant issue for dynamic chambers used in hot regions; however, the reactive losses of highly reactive compounds must still be carefully considered to ensure accurate measurement of their emission rates.
Although species-specific variabilities in adsorptive and reactive losses existed, we demonstrated that MTs in group 1, including α-pinene, β-pinene, 3-carene, limonene, and 1,8-cineole, exhibit consistent adsorptive behavior with α-pinene-d3, regardless of changes in concentration, temperature, or humidity, and the reactive losses of these MTs are minimally affected by ambient-level O3. This consistency makes α-pinene-d3 a suitable surrogate. Similarly, β-caryophyllene-d2 aligns well with β-caryophyllene and α-humulene and is a suitable surrogate for these compounds. However, MTs in group 2 and SQTs in group 1 exhibit significant differences in adsorptive and reactive behaviors compared to α-pinene-d3 and β-caryophyllene-d2, respectively, and therefore cannot be calibrated using these two deuterated surrogates. Given the strong correlation between adsorptive capacity and O3 reactivity (Fig. 7), we recommend considering other deuterated MTs and SQTs that share similar adsorptive and reactive behaviors with those in group 2 and SQTs in group 1 as internal surrogates for loss correction.
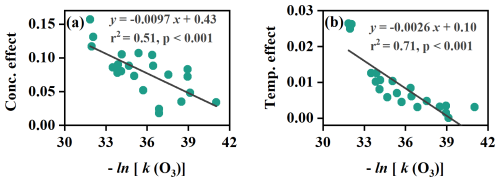
Figure 7Relationship of ozone reactivity with the effects of concentration (a) and temperature (b). The “Conc. effect” represents the absolute change of adsorptive loss per ppb () by fitting the loss ratio and the total mixing ratios of MTs or SQTs using ln(x)+b as in Fig. 6. The “Temp. effect” represents the absolute change of adsorptive loss per degree Celsius () by fitting the loss ratio and the temperature using as in Fig. 6. Each point represents an individual MT or SQT species.
In this study, lab evaluations identified significant species-specific variability in the adsorptive and reactive losses of MTs and SQTs in dynamic chambers, influenced by their distinct physicochemical properties. Adsorptive losses were notably influenced by concentration and temperature, with pronounced variability at low concentrations and low temperatures. The reactive losses were also species-specific, with highly reactive compounds like β-caryophyllene and α-humulene exhibiting significant losses. This emphasizes the importance of using O3-free circulating air during emission measurement to minimize these losses for highly reactive SQTs.
This study supports the use of α-pinene-d3 and β-caryophyllene-d2 as effective surrogates for loss correction. These surrogates showed consistent adsorptive and reactive behaviors with corresponding target compounds (e.g., α-pinene, β-pinene, 3-carene, limonene, and 1,8-cineole for MTs and β-caryophyllene and α-humulene for SQTs), enabling more accurate calibration of losses during in situ measurements. Additionally, the observed correlation between adsorptive capacity and ozone reactivity indicates that other deuterated MTs and SQTs could be employed to expand coverage for loss correction.
Our study demonstrates that adsorptive losses in dynamic chambers are highly temperature dependent. In tropical and subtropical regions, higher temperatures potentially reduce adsorptive losses of MTs and SQTs to acceptable levels. Conversely, low temperatures typical of high-latitude regions could lead to significant adsorptive losses, particularly for SQTs, necessitating careful correction.
To ensure accurate emission measurements, it is crucial to account for both adsorptive and reactive losses of MTs and SQTs, especially those with high reactivity. For highly reactive SQTs like β-caryophyllene and α-humulene, O3-free circulating air should be used to avoid substantial reactive losses. Furthermore, selecting internal surrogates that closely match the adsorptive and reactive properties of target compounds is vital for precise loss correction.
It is important to note that, in addition to adsorptive losses on chamber walls, deuterated surrogates may also be adsorbed on plant surfaces (especially leaf surfaces of broad-leaf species) through passive deposition or active uptake. The relative contributions of wall and plant surface losses to the adsorptive loss are not always known, which may limit the applicability of deuterium-labeled compounds for assessing adsorptive losses. However, a larger wall-to-plant surface area ratio and shorter residence times in the chamber (Zeng et al., 2022a) would make the surrogate method more applicable.
All data used in this study are provided in the manuscript and/or the Supplement.
The supplement related to this article is available online at https://doi.org/10.5194/amt-18-1811-2025-supplement.
JZ designed and carried out the experiments with the support of XW, YZ, WS, and HG. JZ, HR, WP, and ZM analyzed the samples in the lab. JQZ analyzed the data and prepared the original manuscript. XMW and YLZ revised the manuscript.
The contact author has declared that none of the authors has any competing interests.
Publisher's note: Copernicus Publications remains neutral with regard to jurisdictional claims made in the text, published maps, institutional affiliations, or any other geographical representation in this paper. While Copernicus Publications makes every effort to include appropriate place names, the final responsibility lies with the authors.
This work was supported by the National Natural Science Foundation of China (grant no. 42321003), the National Key Research and Development Program (grant nos. 2022YFC3701103 and 2024YFC3714300), the Department of Science and Technology of Guangdong (grant nos. 2023B0303000007 and 2023B1212060049), and the Guangzhou Municipal Science and Technology Bureau (grant no. 202206010057).
This paper was edited by Keding Lu and reviewed by Tao Li and one anonymous referee.
Atkinson, R.: Gas-phase tropospheric chemistry of volatile organic compounds: 1. Alkanes and alkenes, J. Phys. Chem. Ref. Data, 26, 215–290, https://doi.org/10.1063/1.556012, 1997.
Atkinson, R. and Arey, J.: Gas-phase tropospheric chemistry of biogenic volatile organic compounds: a review, Atmos. Environ., 37, S197–S219, https://doi.org/10.1016/s1352-2310(03)00391-1, 2003.
Bourtsoukidis, E., Bonn, B., Dittmann, A., Hakola, H., Hellén, H., and Jacobi, S.: Ozone stress as a driving force of sesquiterpene emissions: a suggested parameterisation, Biogeosciences, 9, 4337–4352, https://doi.org/10.5194/bg-9-4337-2012, 2012.
Di Carlo, P., Brune, W. H., Martinez, M., Harder, H., Lesher, R., Ren, X. R., Thornberry, T., Carroll, M. A., Young, V., Shepson, P. B., Riemer, D., Apel, E., and Campbell, C.: Missing OH reactivity in a forest: Evidence for unknown reactive biogenic VOCs, Science, 304, 722–725, https://doi.org/10.1126/science.1094392, 2004.
Doughty, C. E., Keany, J. M., Wiebe, B. C., Rey-Sanchez, C., Carter, K. R., Middleby, K. B., Cheesman, A. W., Goulden, M. L., da Rocha, H. R., Miller, S. D., Malhi, Y., Fauset, S., Gloor, E., Slot, M., Oliveras Menor, I., Crous, K. Y., Goldsmith, G. R., and Fisher, J. B.: Tropical forests are approaching critical temperature thresholds, Nature, 621, 105–111, https://doi.org/10.1038/s41586-023-06391-z, 2023.
Duhl, T. R., Helmig, D., and Guenther, A.: Sesquiterpene emissions from vegetation: a review, Biogeosciences, 5, 761–777, https://doi.org/10.5194/bg-5-761-2008, 2008.
Edwards, P. M., Aikin, K. C., Dube, W. P., Fry, J. L., Gilman, J. B., de Gouw, J. A., Graus, M. G., Hanisco, T. F., Holloway, J., Hübler, G., Kaiser, J., Keutsch, F. N., Lerner, B. M., Neuman, J. A., Parrish, D. D., Peischl, J., Pollack, I. B., Ravishankara, A. R., Roberts, J. M., Ryerson, T. B., Trainer, M., Veres, P. R., Wolfe, G. M., Warneke, C., and Brown, S. S.: Transition from high- to low-NOx control of night-time oxidation in the southeastern US, Nat. Geosci., 10, 490–495, https://doi.org/10.1038/ngeo2976, 2017.
Feng, Z., Yuan, X., Fares, S., Loreto, F., Li, P., Hoshika, Y., and Paoletti, E.: Isoprene is more affected by climate drivers than monoterpenes: A meta-analytic review on plant isoprenoid emissions, Plant Cell Environ., 42, 1939–1949, https://doi.org/10.1111/pce.13535, 2019.
Ghirardo, A., Lindstein, F., Koch, K., Buegger, F., Schloter, M., Albert, A., Michelsen, A., Winkler, J. B., Schnitzler, J. P., and Rinnan, R.: Origin of volatile organic compound emissions from subarctic tundra under global warming, Glob. Chang. Biol., 26, 1908–1925, https://doi.org/10.1111/gcb.14935, 2020.
Guenther, A. B., Jiang, X., Heald, C. L., Sakulyanontvittaya, T., Duhl, T., Emmons, L. K., and Wang, X.: The Model of Emissions of Gases and Aerosols from Nature version 2.1 (MEGAN2.1): an extended and updated framework for modeling biogenic emissions, Geosci. Model Dev., 5, 1471–1492, https://doi.org/10.5194/gmd-5-1471-2012, 2012.
Guenther, A., Karl, T., Harley, P., Wiedinmyer, C., Palmer, P. I., and Geron, C.: Estimates of global terrestrial isoprene emissions using MEGAN (Model of Emissions of Gases and Aerosols from Nature), Atmos. Chem. Phys., 6, 3181–3210, https://doi.org/10.5194/acp-6-3181-2006, 2006.
He, L., Liu, W., Li, Y., Wang, J., Kuwata, M., and Liu, Y.: Wall loss of semi-volatile organic compounds in a Teflon bag chamber for the temperature range of 262–298 K: mechanistic insight on temperature dependence, Atmos. Meas. Tech., 17, 755–764, https://doi.org/10.5194/amt-17-755-2024, 2024.
He, Q., Tomaz, S., Li, C., Zhu, M., Meidan, D., Riva, M., Laskin, A., Brown, S. S., George, C., Wang, X., and Rudich, Y.: Optical properties of secondary organic aerosol produced by nitrate radical oxidation of biogenic volatile organic compounds, Environ. Sci. Technol., 55, 2878–2889, https://doi.org/10.1021/acs.est.0c06838, 2021.
Helin, A., Hakola, H., and Hellén, H.: Optimisation of a thermal desorption–gas chromatography–mass spectrometry method for the analysis of monoterpenes, sesquiterpenes and diterpenes, Atmos. Meas. Tech., 13, 3543–3560, https://doi.org/10.5194/amt-13-3543-2020, 2020.
Hellén, H., Praplan, A. P., Tykkä, T., Helin, A., Schallhart, S., Schiestl-Aalto, P. P., Bäck, J., and Hakola, H.: Sesquiterpenes and oxygenated sesquiterpenes dominate the VOC (C5–C20) emissions of downy birches, Atmos. Chem. Phys., 21, 8045–8066, https://doi.org/10.5194/acp-21-8045-2021, 2021.
Helmig, D., Bocquet, F., Pollmann, J., and Revermann, T.: Analytical techniques for sesquiterpene emission rate studies in vegetation enclosure experiments, Atmos. Environ., 38, 557–572, https://doi.org/10.1016/j.atmosenv.2003.10.012, 2004.
Helmig, D., Ortega, J., Duhl, T., Tanner, D., Guenther, A., Harley, P., Wiedinmyer, C., Milford, J., and Sakulyanontvittaya, T.: Sesquiterpene emissions from pine trees – Identifications, emission rates and flux estimates for the contiguous United States, Environ. Sci. Technol., 41, 1545–1553, https://doi.org/10.1021/es0618907, 2007.
Helmig, D., Daly, R. W., Milford, J., and Guenther, A.: Seasonal trends of biogenic terpene emissions, Chemosphere, 93, 35–46, https://doi.org/10.1016/j.chemosphere.2013.04.058, 2013.
Hoffmann, T., Odum, J. R., Bowman, F., Collins, D., Klockow, D., Flagan, R. C., and Seinfeld, J. H.: Formation of organic aerosols from the oxidation of biogenic hydrocarbons, J. Atmos. Chem., 26, 189–222, https://doi.org/10.1023/a:1005734301837, 1997.
Hohaus, T., Kuhn, U., Andres, S., Kaminski, M., Rohrer, F., Tillmann, R., Wahner, A., Wegener, R., Yu, Z., and Kiendler-Scharr, A.: A new plant chamber facility, PLUS, coupled to the atmosphere simulation chamber SAPHIR, Atmos. Meas. Tech., 9, 1247–1259, https://doi.org/10.5194/amt-9-1247-2016, 2016.
Jardine, K., Serrano, A. Y., Arneth, A., Abrell, L., Jardine, A., van Haren, J., Artaxo, P., Rizzo, L. V., Ishida, F. Y., Karl, T., Kesselmeier, J., Saleska, S., and Huxman, T.: Within-canopy sesquiterpene ozonolysis in Amazonia, J. Geophys. Res.-Atmos., 116, D19301, https://doi.org/10.1029/2011jd016243, 2011.
Kolari, P., Back, J., Taipale, R., Ruuskanen, T. M., Kajos, M. K., Rinne, J., Kulmala, M., and Hari, P.: Evaluation of accuracy in measurements of VOC emissions with dynamic chamber system, Atmos. Environ., 62, 344–351, https://doi.org/10.1016/j.atmosenv.2012.08.054, 2012.
Kuhn, U., Rottenberger, S., Biesenthal, T., Wolf, A., Schebeske, G., Ciccioli, P., Brancaleoni, E., Frattoni, M., Tavares, T. M., and Kesselmeier, J.: Isoprene and monoterpene emissions of Amazonian tree species during the wet season: Direct and indirect investigations on controlling environmental functions, J. Geophys. Res.-Atmos., 107, 8071, https://doi.org/10.1029/2001jd000978, 2002.
Lu, X., Hong, J., Zhang, L., Cooper, O. R., Schultz, M. G., Xu, X., Wang, T., Gao, M., Zhao, Y., and Zhang, Y.: Severe surface ozone pollution in China: A global perspective, Environ. Sci. Technol. Lett., 5, 487–494, https://doi.org/10.1021/acs.estlett.8b00366, 2018.
Materic, D., Bruhn, D., Turner, C., Morgan, G., Mason, N., and Gauci, V.: Methods in plant foliar volatile organic compounds research, Appl. Plant Sci., 3, 1500044, https://doi.org/10.3732/apps.1500044, 2015.
Niinemets, U., Loreto, F., and Reichstein, M.: Physiological and physicochemical controls on foliar volatile organic compound emissions, Trends Plant Sci., 9, 180–186, https://doi.org/10.1016/j.tplants.2004.02.006, 2004.
Niinemets, Ü., Kuhn, U., Harley, P. C., Staudt, M., Arneth, A., Cescatti, A., Ciccioli, P., Copolovici, L., Geron, C., Guenther, A., Kesselmeier, J., Lerdau, M. T., Monson, R. K., and Peñuelas, J.: Estimations of isoprenoid emission capacity from enclosure studies: measurements, data processing, quality and standardized measurement protocols, Biogeosciences, 8, 2209–2246, https://doi.org/10.5194/bg-8-2209-2011, 2011.
Ortega, J. and Helmig, D.: Approaches for quantifying reactive and low-volatility biogenic organic compound emissions by vegetation enclosure techniques – part A, Chemosphere, 72, 343-364, https://doi.org/10.1016/j.chemosphere.2007.11.020, 2008.
Ortega, J., Helmig, D., Daly, R. W., Tanner, D. M., Guenther, A. B., and Herrick, J. D.: Approaches for quantifying reactive and low-volatility biogenic organic compound emissions by vegetation enclosure techniques – Part B: Applications, Chemosphere, 72, 365–380, https://doi.org/10.1016/j.chemosphere.2008.02.054, 2008.
Peñuelas, J. and Staudt, M.: BVOCs and global change, Trends Plant Sci., 15, 133–144, https://doi.org/10.1016/j.tplants.2009.12.005, 2010.
Pollmann, J., Ortega, J., and Helmig, D.: Analysis of atmospheric sesquiterpenes: Sampling losses and mitigation of ozone interferences, Environ. Sci. Technol., 39, 9620–9629, https://doi.org/10.1021/es050440w, 2005.
Ruuskanen, T. M., Kolari, P., Back, J., Kulmala, M., Rinne, J., Hakola, H., Taipale, R., Raivonen, M., Altimir, N., and Hari, P.: On-line field measurements of monoterpene emissions from Scots pine by proton-transfer-reaction mass spectrometry, Boreal Environ. Res., 10, 553–567, 2005.
Schaub, A., Blande, J. D., Graus, M., Oksanen, E., Holopainen, J. K., and Hansel, A.: Real-time monitoring of herbivore induced volatile emissions in the field, Physiol. Plant., 138, 123–133, https://doi.org/10.1111/j.1399-3054.2009.01322.x, 2010.
Scott, C. E., Arnold, S. R., Monks, S. A., Asmi, A., Paasonen, P., and Spracklen, D. V.: Substantial large-scale feedbacks between natural aerosols and climate, Nat. Geosci., 11, 44–48, https://doi.org/10.1038/s41561-017-0020-5, 2017.
Šimpraga, M., Ghimire, R. P., Van Der Straeten, D., Blande, J. D., Kasurinen, A., Sorvari, J., Holopainen, T., Adriaenssens, S., Holopainen, J. K., and Kivimäenpää, M.: Unravelling the functions of biogenic volatiles in boreal and temperate forest ecosystems, Eur. J. For. Res., 138, 763–787, https://doi.org/10.1007/s10342-019-01213-2, 2019.
Unger, N.: Human land-use-driven reduction of forest volatiles cools global climate, Nat. Clim. Chang., 4, 907–910, https://doi.org/10.1038/nclimate2347, 2014.
Wang, T., Dai, J., Lam, K. S., Nan Poon, C., and Brasseur, G. P.: Twenty-five years of lower tropospheric ozone observations in tropical east Asia: The influence of emissions and weather patterns, Geophys. Res. Lett., 46, 11463–11470, https://doi.org/10.1029/2019gl084459, 2019.
Yli-Juuti, T., Mielonen, T., Heikkinen, L., Arola, A., Ehn, M., Isokaanta, S., Keskinen, H. M., Kulmala, M., Laakso, A., Lipponen, A., Luoma, K., Mikkonen, S., Nieminen, T., Paasonen, P., Petaja, T., Romakkaniemi, S., Tonttila, J., Kokkola, H., and Virtanen, A.: Significance of the organic aerosol driven climate feedback in the boreal area, Nat. Commun., 12, 5637, https://doi.org/10.1038/s41467-021-25850-7, 2021.
Zeng, J., Zhang, Y., Zhang, H., Song, W., Wu, Z., and Wang, X.: Design and characterization of a semi-open dynamic chamber for measuring biogenic volatile organic compound (BVOC) emissions from plants, Atmos. Meas. Tech., 15, 79–93, https://doi.org/10.5194/amt-15-79-2022, 2022a.
Zeng, J., Song, W., Zhang, Y., Mu, Z., Pang, W., Zhang, H., and Wang, X.: Emissions of isoprenoids from dominant tree species in subtropical China, Front. For. Glob. Change, 5, 1089676, https://doi.org/10.3389/ffgc.2022.1089676, 2022b.
Zeng, J., Zhang, Y., Mu, Z., Pang, W., Zhang, H., Wu, Z., Song, W., and Wang, X.: Temperature and light dependency of isoprene and monoterpene emissions from tropical and subtropical trees: Field observations in south China, Appl. Geochem., 155, 105727, https://doi.org/10.1016/j.apgeochem.2023.105727, 2023.
Zeng, J., Zhang, Y., Pang, W., Ran, H., Guo, H., Song, W., and Wang, X.: Optimizing in-situ measurement of representative BVOC emission factors considering intraspecific variability, Geophys. Res. Lett., 51, e2024GL108870, https://doi.org/10.1029/2024gl108870, 2024.
Zhang, Y., Han, Z., Li, X., Zhang, H., Yuan, X., Feng, Z., Wang, P., Mu, Z., Song, W., Blake, D. R., Ying, Q., George, C., Sheng, G., Peng, P. a., and Wang, X.: Plants and related carbon cycling under elevated ground-level ozone: A mini review, Appl. Geochem., 144, 105400, https://doi.org/10.1016/j.apgeochem.2022.105400, 2022.