the Creative Commons Attribution 4.0 License.
the Creative Commons Attribution 4.0 License.
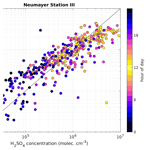
Estimation of sulfuric acid concentration using ambient ion composition and concentration data obtained with atmospheric pressure interface time-of-flight ion mass spectrometer
Siegfried Schobesberger
Mikko Sipilä
Veli-Matti Kerminen
Markku Kulmala
Sulfuric acid (H2SO4, SA) is the key compound in atmospheric new particle formation. Therefore, it is crucial to observe its concentration with sensitive instrumentation, such as chemical ionisation (CI) inlets coupled to atmospheric pressure interface time-of-flight (APi-TOF) mass spectrometers. However, there are environmental conditions for which and physical reasons why chemical ionisation cannot be used, for example in certain remote places or during flight measurements with limitations regarding chemicals. Here, we propose a theoretical method to estimate the SA concentration based on ambient ion composition and concentration measurements that are achieved by APi-TOF alone. We derive a theoretical expression to estimate the SA concentration and validate it with accurate CI-APi-TOF observations. Our validation shows that the developed estimate works well during daytime in a boreal forest (R2=0.85); however, it underestimates the SA concentration in, e.g. the Antarctic atmosphere during new particle formation events where the dominating pathway for nucleation involves sulfuric acid and a base (R2=0.48).
- Article
(4705 KB) - Full-text XML
- BibTeX
- EndNote
Sulfuric acid (H2SO4, SA) is the key compound in atmospheric new particle formation (e.g. Weber et al., 1995, 1996; Birmili et al., 2003; Kulmala et al., 2004, 2014; Kuang et al., 2008; Kerminen et al., 2010; Wang et al., 2011; Yao et al., 2018; Cai et al., 2021); therefore, it is crucial to have accurate observations of its concentration. However, ambient concentrations of H2SO4 are low, commonly less than a part per trillion by volume ( molecules cm−3), making it challenging to measure it. During the recent years there have been instrumental developments towards a reliable detection of H2SO4 in the atmosphere, particularly via the development of a chemical ionisation atmospheric pressure interface time-of-flight (CI-APi-TOF) mass spectrometer (Jokinen et al., 2012), using nitrate as a reagent ion. Still, the measurement technique with CI-APi-TOF is relatively challenging, as a thorough calibration, i.e. with sulfuric acid as proposed by Kürten et al. (2012), is needed in order to get reliable numbers. Furthermore, the loss of sulfuric acid to surfaces, such as an inlet, and the correct flow rates must be known and characterised.
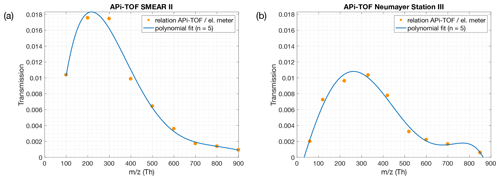
Figure 1Ion transmission of the APi-TOF mass spectrometers used in this study. The transmission efficiency was determined via production of charged particles with a NiCr wire. The concentration of the size-selected ions with a Hermann nano differential mobility analyser (HDMA; Hermann, 2000) were measured with an electrometer and an APi-TOF in parallel. A more detailed description can be found in Junninen et al. (2010). Panel (a) shows the transmission efficiency of the APi-TOF used for measurements at the SMEAR II station, Hyytiälä, Finland. Panel (b) shows the transmission efficiency used for measurements at Neumayer Station III.
During the past decade, APi-TOF mass spectrometers (Junninen et al., 2010) have been deployed in several measurement campaigns where the use of a CI inlet was either not possible or desired. In these instances, the APi-TOF only observed the composition and concentration of ambient ions. The APi-TOF is capable of directly sampling and detecting naturally charged gas-phase ions, including molecular clusters, and is often used to detect clustering processes as a first step of new particle formation on a molecular basis (e.g. Schobesberger et al., 2013; Jokinen et al., 2018; Beck et al., 2021a). While a CI-APi-TOF has a limit of detection of, at best, around 104 molecules cm−3 (∼ ppq level), the APi-TOF can detect approximately 1 % of the ambient ion concentration (Fig. 1; Junninen et al., 2010). With an average ion concentration of ∼1000 cm−3 per polarity (Hirsikko et al., 2011), the APi-TOF measures 10 ions cm−3 s−1 with a limit of detection of ∼0.01 counts per second, hence 0.1 ions cm−3. This approximately corresponds to parts per sextillion (), showing that the limit of detection of an APi-TOF in comparison to a CI-APi-TOF is lower by 5 orders of magnitude.
A detailed description of the APi-TOF can be found in Junninen et al. (2010). Since concentrations of neutral clusters are below the detection limit of CI-APi-TOF in many atmospheric conditions and environments, using the APi-TOF is currently the only way to directly detect atmospheric clustering. Therefore, if we can estimate H2SO4 concentration particularly during initial steps of new particle formation, based on the same dataset, we can readily get better insight into the process itself.
Since there are only limited long-term observations of H2SO4 concentrations, several proxies have been developed (e.g. Petäjä et al., 2009; Mikkonen et al., 2011; Lu et al., 2019; Dada et al., 2020). These proxies attempt to approximate the ambient H2SO4 concentrations using more readily measured quantities, in particular the sulfur dioxide concentration, (UV) radiation intensity and pre-existing particle number size distribution that can be used to calculate the condensation sink for gas-phase H2SO4. In circumstances where the required data for H2SO4 proxies are not available, but APi-TOF measurements have been conducted, the H2SO4 concentration can be obtained from the ion mass spectra. The first attempt to estimate sulfuric acid concentration via the concentration of atmospheric ions was taken by Arnold and Fabian (1980); this was followed by Eisele (1989) under the assumption that most H2SO4 molecules are charged by reacting with NO.
Motivated by the reasonings outlined above, we derive here an expression to estimate H2SO4 concentration based primarily on APi-TOF observations and then validate it.
Ambient ion mass spectra usually show clear evidence of gas-phase H2SO4, predominantly in the form of bisulfate ions () and their adducts involving H2SO4, forming dimers , as well as larger clusters (Ehn et al., 2010). These ions' existence is due to the efficient scavenging of a negative charge by ambient H2SO4 via proton donation, and due to the high stability of the sulfuric acid–bisulfate ion clusters, in particular for the dimer (Ortega et al., 2014). In order to estimate the sulfuric acid concentration (H2SO4) using measured naturally charged ions (see Fig. 2), we approximate this concentration by following the bisulfate ion , herein denoted SAmonomer, the dimer cluster (SAdimer) and trimer cluster (SAtrimer). Any other H2SO4-containing ion clusters, in particular those larger than SAtrimer, typically occur at much smaller concentrations and will be neglected here.
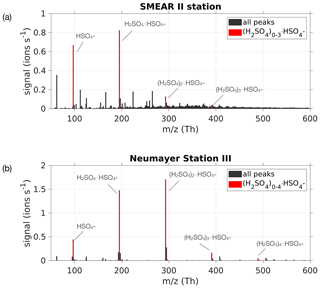
Figure 2(a) Mass spectrum from 50 to 600 Th measured with the APi-TOF on 24 May 2017 during the time period 08:00–18:00 LT at the SMEAR II station, Hyytiälä, Finland. (b) Mass spectrum from 14 January 2019 between 08:00 and 18:00 LT at Neumayer Station III, Antarctica, during a new particle formation event. The bisulfate ion and H2SO4 clusters containing it were used for the estimation of H2SO4 concentration, and are coloured in red.
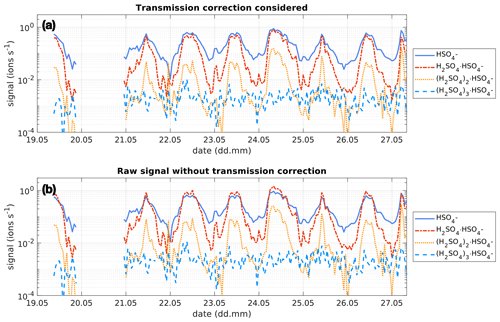
Figure 3Time series of the bisulfate ion (HSO, SAmonomer), H2SO4 clustered with bisulfate (H, SAdimer), two H2SO4 molecules clustered with the bisulfate ion ((H2SO4)2⋅, SAtrimer) and three H2SO4 molecules clustered with the bisulfate ion ((H2SO4)3⋅, SAtetramer) between 19 and 27 May 2017 at the SMEAR II station, Hyytiälä, Finland. The concentration is given in ions s−1 as measured by the APi-TOF. Panel (a) shows the concentration of the clusters considering the transmission efficiency of the instrument (see Fig. 1). Panel (b) shows the concentration of the clusters without that correction and assuming a constant transmission efficiency of 1 % for all ions.
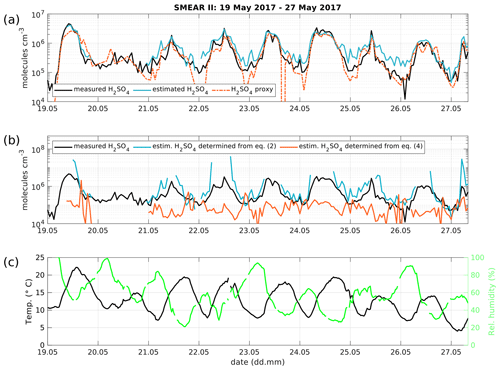
Figure 4(a) Time series of measured H2SO4 concentration from the CI-APi-TOF (black) and estimated H2SO4 concentration from the APi-TOF (blue) and H2SO4 proxy from Dada et al. (2020; orange) between 19 and 27 May 2017. The concentration is given in molecules cm−3. (b) Measured H2SO4 concentration as in panel (a) in black and determined concentration from Eq. (2) (blue) and Eq. (4) (orange). (c) Temperature and relative humidity.
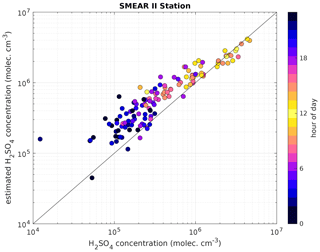
Figure 5Measured H2SO4 concentration using a CI-APi-TOF (horizontal axis) versus estimated H2SO4 concentration based on APi-TOF results (vertical axis) at the SMEAR II station. For the estimation of H2SO4, the transmission efficiency was taken into account. The colour indicates the hour of the day and the black line is the 1:1 ratio. Between 08:00 and 16:00 LT, the concentrations agree well. The data shown cover the time period from 19 to 27 May 2017. The overall correlation coefficient (Pearson) is 0.94.
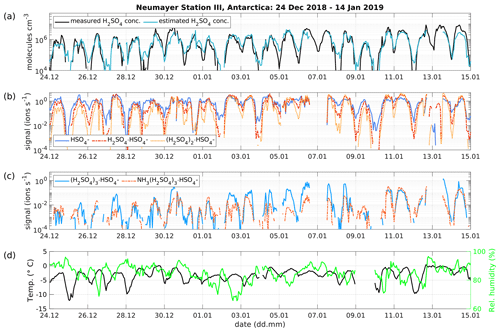
Figure 6(a) Time series of measured H2SO4 concentration from the CI-APi-TOF (black) and estimated H2SO4 concentration from the APi-TOF (blue) between 24 December 2018 and 14 January 2019 at Neumayer Station III, Antarctica. The concentration is given in molecules cm−3. (b) Time series of the bisulfate ion (HSO4−, SAmonomer), H2SO4 clustered with bisulfate (H2SO, SAdimer), two H2SO4 molecules clustered with the bisulfate ion ((H, SAtrimer) and (c), three H2SO4 molecules clustered with the bisulfate ion ((H, SAtetramer) and the SAtetramer clustered with NH3. (d) Temperature and relative humidity measured at Neumayer Station III.
If we assume that the concentration of SAmonomer depends generally on its production rate (P1) and that its loss is by condensation onto aerosol particles (condensation sink, CS), to the SAdimer when clustering with another H2SO4 molecule, and to ion–ion recombination with positive ions (Npos), we get the following equation for the SAmonomer concentration:
where ] is the dimer production rate from SAmonomer–H2SO4 collisions, α ( cm3 s−1) is the ion–ion recombination coefficient (Kontkanen et al., 2013) and the collision rate k1 is assumed to be constant.
For the dimer concentration we consider the production P2, the loss due to CS, the clustering of the SAdimer with H2SO4 with a rate constant k2 and the ion–ion recombination:
and with substituting P2, Eq. (2) for SAdimer changes to
Finally, to produce SAtrimer we consider the collision of the SAdimer with H2SO4 and the loss to the CS and ion–ion recombination. For the sake of completeness, we would additionally have to consider the loss of SAtrimers to form the tetramer (H2SO4)3 ⋅ HSO4; however, this additional term is rather small and will therefore be neglected in this derivation. Therefore, we get the simplified equation for SAtrimer:
For simplification, we consider a pseudo-steady-state condition for both dimers and trimers by setting the left-hand side of Eqs. (3) and (4) to be zero, which is justified when the dimer and trimer concentrations change at rates smaller than their overall production and loss rates. Thereby, from Eq. (3) we obtain
and from Eq. (4) we obtain
If we now deploy Eq. (6) in Eq. (5) and solve for H2SO4, the result is
Besides the steady-state assumption, it should be noted that in deriving Eq. (8), monomers, dimers and trimers were assumed to have the same loss rate (CS) onto pre-existing aerosol particles. This causes an additional, yet minor, uncertainty in the estimated H2SO4 concentrations, as such loss rates are dependent on the size/mass of the clusters (e.g. Lehtinen et al., 2007; Tuovinen et al., 2021). According to Tuovinen et al. (2021), the CS of H2SO4 clusters decreases with an increasing number of H2SO4 molecules. The study shows that the CS of the SAdimer clustered with ammonia decreases to 68 % (compared to one H2SO4 molecule) and for SApentamer with four ammonia molecules to 42 %. However, the order of magnitude of the CS remains the same, and the effect on the estimation of the H2SO4 concentration is assumed to be negligible. Additionally, the CS for ions is higher than for neutral compounds. The enhancement of CS has been shown by Mahfouz and Donahue (2021) to reach a maximum value of 2 when the pre-existing particles are <10 nm and to decrease to 1 when the pre-existing particles are >100 nm. The impact of ions on CS and estimated SA concentrations depends thereby on the environmental conditions determining the size distribution and charges of the pre-existing particle population. Neglecting the size dependency of CS between the SA monomers, dimers and trimers causes additional errors in the estimated SA concentrations; however, it is difficult to determine this effect in ambient measurements with limited data and instrumentation.
Table 1Root mean square error (RMSE) and R2 of the estimated H2SO4 concentration at the SMEAR II station and Neumayer Station III. Daytime and nighttime are split as 06:00–18:00 LT and 18:00–06:00 LT respectively. For the SMEAR II station, we also show the RMSE and R2 of the H2SO4 proxy calculated with the method introduced by Dada et al. (2020).
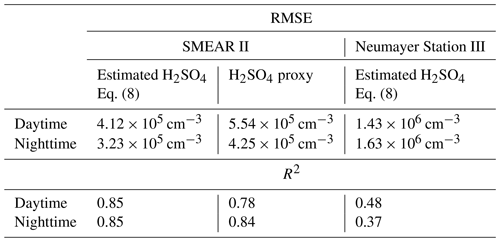
Furthermore, the derivation neglects the losses of SAtrimer to the SAtetramer and larger clusters, as well as the clustering of sulfuric acid ion clusters with water and base molecules, such as NH3. These simplifications can cause an underestimation of the H2SO4 concentration with the presented method. If necessary, the method can easily be adapted, and bigger clusters can be included in the equation.
From Eq. (8) we also see that the concentration of H2SO4 is proportional to relative concentrations of sulfuric acid monomers, dimers and trimers clustered with the bisulfate ion:
To estimate the H2SO4 concentration with the “ion mode” APi-TOF, we can therefore use this theoretical approach, in particular Eq. (8). For the collision rate of H2SO4 with , we use cm3 molecule−1 s−1 as in Lovejoy et al. (2004). The value of CS is calculated based on Kulmala et al. (2012). Even if the CS was unknown due, for example, to the lack of particle measurements, the daytime variability of the H2SO4 concentration could still be estimated by using the relation of the H2SO4-containing cluster with , as it is proportional to the H2SO4 concentration (see Eq. 9). If the concentration of positive small ions is not available, it can be assumed to be in the range of 500–1000 cm−3, which is a reasonable approximation for the average concentration (Hirsikko et al., 2011).
As the transmission of clusters within an APi-TOF depends on the tuning of the instrument and on the pressures within its chambers, the transmission efficiency needs to be considered, in order to get reliable concentrations of the SAmonomer, SAdimer and SAtrimer. Figure 1 shows the transmission efficiency curve of the APi-TOF used at the SMEAR II station and Neumayer Station III. The effect of applying the transmission correction to the different SA clusters is depicted in Fig. 3 for the time series at the SMEAR II station. All ion signals were normalised to a transmission of 1 %. As can be determined from Fig. 1a, the SAmonomer transmission at SMEAR II was ∼1 %, while the dimer and trimer were corrected by a factor of and respectively. The correction was also applied to the ions measured at Neumayer Station III according to the APi-TOF transmission (Fig. 1b).
We tested the expression derived above using a dataset collected during inter-comparison measurements at the SMEAR II station in Hyytiälä, Finland (Hari and Kulmala, 2005). In Fig. 4 we show the time series of the observed H2SO4 concentrations, measured with a CI-APi-TOF. The CI-APi-TOF was calibrated for sulfuric acid, based on the method by Kürten et al. (2012) and resulted in a calibration factor of 2.5×109 cm−3. Additionally, we show the estimated sulfuric acid concentration based on APi-TOF measurements together with Eq. (8) and the sulfuric acid proxy concentration (Dada et al., 2020). The concentration of positive ions for the estimated sulfuric acid concentration was obtained from a neutral cluster and air ion spectrometer (NAIS, Airel Ltd.; Mirme and Mirme, 2013).
The estimated H2SO4 concentration agrees with that measured during most of the daytime (between 06:00 and 18:00 LT), during which the correlation (R2) between the estimated and measured H2SO4 concentration is equal to 0.85, with a root mean square error (RMSE) of 4.12×105 cm−3. During nighttime, the corresponding values are 0.85 and 3.23×105 cm−3 (Table 1).
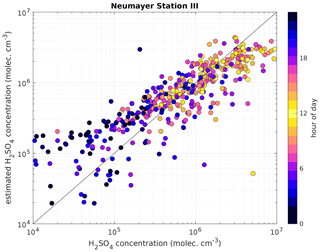
Figure 7Measured H2SO4 concentration using a CI-APi-TOF (horizontal axis) versus estimated H2SO4 concentration based on APi-TOF results (vertical axis) at Neumayer Station III. For the estimation of H2SO4, the transmission efficiency was taken into account. The colour indicates the hour of the day and the black line is the 1:1 ratio. The data shown cover the time period from 24 December 2016 to 14 January 2019. The overall correlation coefficient (Pearson) is 0.77.
The scatter plot in Fig. 5 shows that the estimated H2SO4 concentrations agree well with those observed when they are larger than 2×106 cm−3, demonstrating that our method works particularly well at the SMEAR II station during conditions that favour the formation of H2SO4-containing clusters.
For the sake of completeness, the estimation of the H2SO4 concentration determined from Eqs. (2) and (4), assuming a pseudo-steady state, are depicted in Fig. 4b. The H2SO4 concentration from Eq. (2) is an overestimate, while solving Eq. (4) for H2SO4 underestimates the real concentration as these equations are only approximations. By combining the various approximations, Eq. (8) yields in the best fit to the observed SA concentration.
The presented method was also applied to measurements taken at Neumayer Station III, Antarctica, in order to test it in a different environment. Here, we used the condensation sink reported by Weller et al. (2015) at the Neumayer station of s−1. Figure 6 shows a 3-week period between 24 December 2018 and 14 January 2019. The calibration factor of the CI-APi-TOF used for measuring the sulfuric acid concentration is 4.9×109 cm−3. Here, the sulfuric acid concentration is underestimated versus the measured concentration when the SAtetramer and NH3(H2SO4)3 clusters show high concentrations (Fig. 6c). A possible explanation for the underestimation might be the neglection of the growth of sulfuric acid to oligomers larger than the tetramer, as well as its clustering with bases and water (Fig. 6b and c). In coastal Antarctica, the main nucleating mechanism was observed to be negative ion-induced sulfuric acid–ammonia nucleation, acting as a major sink for sulfuric acid molecules due to its clustering with bases (Jokinen et al., 2018). Including the SAtetramer and SAtetramer clustered with NH3 in the estimation equation improved the correlation (R2) from 0.48 to 0.54. Furthermore, as mentioned above, the value of CS for Neumayer was assumed to be constant (10−3 s−1) due to the lack of data needed for its calculation. This simplification certainly causes additional errors in estimated SA concentrations, especially during periods of high sea salt concentrations causing potentially large variations in values of CS. Nevertheless, the diurnal variation of the SA concentration is represented well by this method. During times with lower sulfuric acid concentrations, our method gives higher values than the measured concentrations (Figs. 6 and 7).
Here we derived a theoretical expression to estimate H2SO4 concentrations based on APi-TOF measurements of ambient ions. The estimated concentration agrees well with the measured concentration during daytime in a boreal forest (R2=0.85), indicating that the estimation is able to represent the diurnal variation and trend of H2SO4 concentrations during most of the time when active clustering of sulfuric acid induces the initial step(s) of atmospheric new particle formation. However, in an atmosphere where sulfuric acid is the dominating pathway for initiating new particle formation, the method might underestimate H2SO4 concentrations as this method does not include rapid clustering to bigger sulfuric acid clusters or clustering with bases directly, e.g. in the Antarctic atmosphere (R2=0.48; during daytime).
The APi-TOF “ion mode”, i.e. direct ion sampling without chemical ionisation, remains a crucial tool in many field deployments and laboratory studies, since it is extremely sensitive and allows for observing atmospheric clustering molecule by molecule, which in most cases is impossible when relying on chemical ionisation. Therefore, having a reliable estimate of H2SO4 concentration available allows us to utilise the APi-TOF ion mode even more effectively.
The data can be accessed via Zenodo (https://doi.org/10.5281/zenodo.5266313; Beck et al., 2021b).
LJB, SiS, VMK and MK designed the study. LJB and MS performed the measurements. SiS and LJB derived the equations. LJB processed and analysed the data and performed the data visualisation. MK and VMK supervised the process. All authors commented and edited the paper.
The contact author has declared that neither they nor their co-authors have any competing interests.
Publisher’s note: Copernicus Publications remains neutral with regard to jurisdictional claims in published maps and institutional affiliations.
We thank the tofTools team for providing the tools for mass spectrometry analysis. We thank the technical and scientific staff of Hyytiälä SMEAR II and the technicians and scientists of the Neumayer overwintering teams of the years 2018 and 2019. We thank Lubna Dada for calculating the SA proxy for the SMEAR II station. We thank Janne Lampilahti for providing the codes to process the NAIS dataset.
This research has been supported by the Academy of Finland (grant nos. 337549, 302958, 1325656, 310682, 316114, 325647 and 296628),
the “Quantifying carbon sink, CarbonSinkC and their interaction with air quality” INAR project funded by the Jane and Aatos Erkko Foundation, the European Research Council (ERC) project ATM-GTP (contract no. 742206) and GASPARCON (grant no. 714621).
Open-access funding was provided by the Helsinki University Library.
This paper was edited by Joachim Curtius and reviewed by two anonymous referees.
Arnold, F. and Fabian, R.: First measurements of gas phase sulphuric acid in the stratosphere, Nature, 283, 55–57, https://doi.org/10.1038/283055a0, 1980.
Beck, L. J., Sarnela, N., Junninen, H., Hoppe, C. J. M., Garmash, O., Bianchi, F., Riva, M., Rose, C., Peräkylä, O., Wimmer, D., Kausiala, O., Jokinen, T., Ahonen, L., Mikkilä, J., Hakala, J., He, X.-C., Kontkanen, J., Wolf, K. K. E., Cappelletti, D., Mazzola, M., Traversi, R., Petroselli, C., Viola, A. P., Vitale, V., Lange, R., Massling, A., Nøjgaard, J. K., Krejci, R., Karlsson, L., Zieger, P., Jang, S., Lee, K., Vakkari, V., Lampilahti, J., Thakur, R. C., Leino, K., Kangasluoma, J., Duplissy, E.-M., Siivola, E., Marbouti, M., Tham, Y. J., Saiz-Lopez, A., Petäjä, T., Ehn, M., Worsnop, D. R., Skov, H., Kulmala, M., Kerminen, V.-M., and Sipilä, M.: Differing Mechanisms of New Particle Formation at Two Arctic Sites, Geophys. Res. Lett., 48, e2020GL091334, https://doi.org/10.1029/2020GL091334, 2021a.
Beck, L. J., Schobesberger, S., Sipilä, M., Kerminen, V.-M., and Kulmala, M.: Estimation of sulfuric acid concentrations using ambient ion composition and concentration data obtained by ion mass spectrometry measurements (APi-TOF), Zenodo [data set], https://doi.org/10.5281/zenodo.5266313, 2021b.
Birmili, W., Berresheim, H., Plass-Dülmer, C., Elste, T., Gilge, S., Wiedensohler, A., and Uhrner, U.: The Hohenpeissenberg aerosol formation experiment (HAFEX): a long-term study including size-resolved aerosol, H2SO4, OH, and monoterpenes measurements, Atmos. Chem. Phys., 3, 361–376, https://doi.org/10.5194/acp-3-361-2003, 2003.
Cai, R., Yan, C., Yang, D., Yin, R., Lu, Y., Deng, C., Fu, Y., Ruan, J., Li, X., Kontkanen, J., Zhang, Q., Kangasluoma, J., Ma, Y., Hao, J., Worsnop, D. R., Bianchi, F., Paasonen, P., Kerminen, V.-M., Liu, Y., Wang, L., Zheng, J., Kulmala, M., and Jiang, J.: Sulfuric acid–amine nucleation in urban Beijing, Atmos. Chem. Phys., 21, 2457–2468, https://doi.org/10.5194/acp-21-2457-2021, 2021.
Dada, L., Ylivinkka, I., Baalbaki, R., Li, C., Guo, Y., Yan, C., Yao, L., Sarnela, N., Jokinen, T., Daellenbach, K. R., Yin, R., Deng, C., Chu, B., Nieminen, T., Wang, Y., Lin, Z., Thakur, R. C., Kontkanen, J., Stolzenburg, D., Sipilä, M., Hussein, T., Paasonen, P., Bianchi, F., Salma, I., Weidinger, T., Pikridas, M., Sciare, J., Jiang, J., Liu, Y., Petäjä, T., Kerminen, V.-M., and Kulmala, M.: Sources and sinks driving sulfuric acid concentrations in contrasting environments: implications on proxy calculations, Atmos. Chem. Phys., 20, 11747–11766, https://doi.org/10.5194/acp-20-11747-2020, 2020.
Ehn, M., Junninen, H., Petäjä, T., Kurtén, T., Kerminen, V.-M., Schobesberger, S., Manninen, H. E., Ortega, I. K., Vehkamäki, H., Kulmala, M., and Worsnop, D. R.: Composition and temporal behavior of ambient ions in the boreal forest, Atmos. Chem. Phys., 10, 8513–8530, https://doi.org/10.5194/acp-10-8513-2010, 2010.
Eisele, F. L.: Natural and anthropogenic negative ions in the troposphere, J. Geophys. Res.-Atmos., 94, 2183–2196, https://doi.org/10.1029/JD094iD02p02183, 1989.
Hari, P. and Kulmala, M.: Station for Measuring Ecosystem–Atmosphere Relations (SMEAR II), Boreal Environ. Res., 10, 315–322, 2005.
Herrmann, W., Eichler, T., Bernardo, N., and Fernandez de la Mora, J.: Turbulent transition arises at Re 35 000 in a short Vienna type DMA with a large laminarizing inlet, in: Proceedings of the annual conference of the AAAR, St. Louis, Missouri, USA, 6–10 October 2000.
Hirsikko, A., Nieminen, T., Gagné, S., Lehtipalo, K., Manninen, H. E., Ehn, M., Hõrrak, U., Kerminen, V.-M., Laakso, L., McMurry, P. H., Mirme, A., Mirme, S., Petäjä, T., Tammet, H., Vakkari, V., Vana, M., and Kulmala, M.: Atmospheric ions and nucleation: a review of observations, Atmos. Chem. Phys., 11, 767–798, https://doi.org/10.5194/acp-11-767-2011, 2011.
Jokinen, T., Sipilä, M., Junninen, H., Ehn, M., Lönn, G., Hakala, J., Petäjä, T., Mauldin III, R. L., Kulmala, M., and Worsnop, D. R.: Atmospheric sulphuric acid and neutral cluster measurements using CI-APi-TOF, Atmos. Chem. Phys., 12, 4117–4125, https://doi.org/10.5194/acp-12-4117-2012, 2012.
Jokinen, T., Sipilä, M., Kontkanen, J., Vakkari, V., Tisler, P., Duplissy, E.-M., Junninen, H., Kangasluoma, J., Manninen, H. E., Petäjä, T., Kulmala, M., Worsnop, D. R., Kirkby, J., Virkkula, A., and Kerminen, V.-M.: Ion-induced sulfuric acid–ammonia nucleation drives particle formation in coastal Antarctica, Sci. Adv., 4, eaat9744, https://doi.org/10.1126/sciadv.aat9744, 2018.
Junninen, H., Ehn, M., Petäjä, T., Luosujärvi, L., Kotiaho, T., Kostiainen, R., Rohner, U., Gonin, M., Fuhrer, K., Kulmala, M., and Worsnop, D. R.: A high-resolution mass spectrometer to measure atmospheric ion composition, Atmos. Meas. Tech., 3, 1039–1053, https://doi.org/10.5194/amt-3-1039-2010, 2010.
Kerminen, V.-M., Petäjä, T., Manninen, H. E., Paasonen, P., Nieminen, T., Sipilä, M., Junninen, H., Ehn, M., Gagné, S., Laakso, L., Riipinen, I., Vehkamäki, H., Kurten, T., Ortega, I. K., Dal Maso, M., Brus, D., Hyvärinen, A., Lihavainen, H., Leppä, J., Lehtinen, K. E. J., Mirme, A., Mirme, S., Hõrrak, U., Berndt, T., Stratmann, F., Birmili, W., Wiedensohler, A., Metzger, A., Dommen, J., Baltensperger, U., Kiendler-Scharr, A., Mentel, T. F., Wildt, J., Winkler, P. M., Wagner, P. E., Petzold, A., Minikin, A., Plass-Dülmer, C., Pöschl, U., Laaksonen, A., and Kulmala, M.: Atmospheric nucleation: highlights of the EUCAARI project and future directions, Atmos. Chem. Phys., 10, 10829–10848, https://doi.org/10.5194/acp-10-10829-2010, 2010.
Kontkanen, J., Lehtinen, K. E. J., Nieminen, T., Manninen, H. E., Lehtipalo, K., Kerminen, V.-M., and Kulmala, M.: Estimating the contribution of ion–ion recombination to sub-2 nm cluster concentrations from atmospheric measurements, Atmos. Chem. Phys., 13, 11391–11401, https://doi.org/10.5194/acp-13-11391-2013, 2013.
Kuang, C., McMurry, P. H., McCormick, A. V., and Eisele, F. L.: Dependence of nucleation rates on sulfuric acid vapor concentration in diverse atmospheric locations, J. Geophys. Res., 113, D10209, https://doi.org/10.1029/2007JD009253, 2008.
Kulmala, M., Vehkamäki, H., Petäjä, T., Dal Maso, M., Lauri, A., Kerminen, V.-M., Birmili, W., and McMurry, P. H.: Formation and growth rates of ultrafine atmospheric particles: a review of observations, J. Aerosol Sci., 35, 143–176, https://doi.org/10.1016/j.jaerosci.2003.10.003, 2004.
Kulmala, M., Petäjä, T., Nieminen, T., Sipilä, M., Manninen, H. E., Lehtipalo, K., Dal Maso, M., Aalto, P. P., Junninen, H., Paasonen, P., Riipinen, I., Lehtinen, K. E. J., Laaksonen, A., and Kerminen, V.-M.: Measurement of the nucleation of atmospheric aerosol particles, Nature, 7, 1651–1667, https://doi.org/10.1038/nprot.2012.091, 2012.
Kulmala, M., Petäjä, T., Ehn, M., Thornton, J., Sipilä, M., Worsnop, D. R., and Kerminen, V.-M.: Chemistry of Atmospheric Nucleation: On the Recent Advances on Precursor Characterization and Atmospheric Cluster Composition in Connection with Atmospheric New Particle Formation, Annu. Rev. Phys. Chem., 65, 21–37, https://doi.org/10.1146/annurev-physchem-040412-110014, 2014.
Kürten, A., Rondo, L., Ehrhart, S., and Curtius, J.: Calibration of a Chemical Ionization Mass Spectrometer for the Measurement of Gaseous Sulfuric Acid, J. Phys. Chem. A, 116, 6375–6386, https://doi.org/10.1021/jp212123n, 2012.
Lehtinen, K. E. J., Dal Maso, M., Kulmala, M., and Kerminen, V.-M.: Estimating nucleation rates from apparent particle formation rates and vice versa: Revised formulation of the Kerminen–Kulmala equation, J. Aerosol Sci., 38, 988–994, https://doi.org/10.1016/j.jaerosci.2007.06.009, 2007.
Lovejoy, E. R., Curtius, J., and Froyd, K. D.: Atmospheric ion-induced nucleation of sulfuric acid and water, J. Geophys. Res., 109, D08204, https://doi.org/10.1029/2003JD004460, 2004.
Lu, Y., Yan, C., Fu, Y., Chen, Y., Liu, Y., Yang, G., Wang, Y., Bianchi, F., Chu, B., Zhou, Y., Yin, R., Baalbaki, R., Garmash, O., Deng, C., Wang, W., Liu, Y., Petäjä, T., Kerminen, V.-M., Jiang, J., Kulmala, M., and Wang, L.: A proxy for atmospheric daytime gaseous sulfuric acid concentration in urban Beijing, Atmos. Chem. Phys., 19, 1971–1983, https://doi.org/10.5194/acp-19-1971-2019, 2019.
Mahfouz, N. G. A. and Donahue, N. M.: Technical note: The enhancement limit of coagulation scavenging of small charged particles, Atmos. Chem. Phys., 21, 3827–3832, https://doi.org/10.5194/acp-21-3827-2021, 2021.
Mikkonen, S., Romakkaniemi, S., Smith, J. N., Korhonen, H., Petäjä, T., Plass-Duelmer, C., Boy, M., McMurry, P. H., Lehtinen, K. E. J., Joutsensaari, J., Hamed, A., Mauldin III, R. L., Birmili, W., Spindler, G., Arnold, F., Kulmala, M., and Laaksonen, A.: A statistical proxy for sulphuric acid concentration, Atmos. Chem. Phys., 11, 11319–11334, https://doi.org/10.5194/acp-11-11319-2011, 2011.
Mirme, S. and Mirme, A.: The mathematical principles and design of the NAIS – a spectrometer for the measurement of cluster ion and nanometer aerosol size distributions, Atmos. Meas. Tech., 6, 1061–1071, https://doi.org/10.5194/amt-6-1061-2013, 2013.
Ortega, I. K., Olenius, T., Kupiainen-Määttä, O., Loukonen, V., Kurtén, T., and Vehkamäki, H.: Electrical charging changes the composition of sulfuric acid–ammonia/dimethylamine clusters, Atmos. Chem. Phys., 14, 7995–8007, https://doi.org/10.5194/acp-14-7995-2014, 2014.
Petäjä, T., Mauldin, III, R. L., Kosciuch, E., McGrath, J., Nieminen, T., Paasonen, P., Boy, M., Adamov, A., Kotiaho, T., and Kulmala, M.: Sulfuric acid and OH concentrations in a boreal forest site, Atmos. Chem. Phys., 9, 7435–7448, https://doi.org/10.5194/acp-9-7435-2009, 2009.
Schobesberger, S., Junninen, H., Bianchi, F., Lönn, G., Ehn, M., Lehtipalo, K., Dommen, J., Ehrhart, S., Ortega, I. K., Franchin, A., Nieminen, T., Riccobono, F., Hutterli, M., Duplissy, J., Almeida, J., Amorim, A., Breitenlechner, M., Downard, A. J., Dunne, E. M., Flagan, R. C., Kajos, M., Keskinen, H., Kirkby, J., Kupc, A., Kürten, A., Kurtén, T., Laaksonen, A., Mathot, S., Onnela, A., Praplan, A. P., Rondo, L., Santos, F. D., Schallhart, S., Schnitzhofer, R., Sipilä, M., Tomé, A., Tsagkogeorgas, G., Vehkamäki, H., Wimmer, D., Baltensperger, U., Carslaw, K. S., Curtius, J., Hansel, A., Petäjä, T., Kulmala, M., Donahue, N. M., and Worsnop, D. R.: Molecular understanding of atmospheric particle formation from sulfuric acid and large oxidized organic molecules, P. Natl. Acad. Sci. USA, 110, 17223–17228, https://doi.org/10.1073/pnas.1306973110, 2013.
Tuovinen, S., Kontkanen, J., Cai, R., and Kulmala, M.: Condensation sink of atmospheric vapors: the effect of vapor properties and the resulting uncertainties, Environ. Sci.-Atmos., 1, 543–557, https://doi.org/10.1039/D1EA00032B, 2021.
Wang, Z. B., Hu, M., Yue, D. L., Zheng, J., Zhang, R. Y., Wiedensohler, A., Wu, Z. J., Nieminen, T., and Boy, M.: Evaluation on the role of sulfuric acid in the mechanisms of new particle formation for Beijing case, Atmos. Chem. Phys., 11, 12663–12671, https://doi.org/10.5194/acp-11-12663-2011, 2011.
Weber, R. J., McMurry, P. H., Eisele, F. L., and Tanner, D. J.: Measurement of Expected Nucleation Precursor Species and 3–500 nm Diameter Particles at Mauna Loa Observatory, Hawaii, J. Atmos. Sci., 52, 2242–2257, 1995.
Weber, R. J., Marti, J. J., McMurry, P. H., Eisele, F. L., Tanner, D. J., and Jefferson, A.: Measured Atmospheric New Particle Formation Rates: Implications for Nucleation Mechanisms, Chem. Eng. Commun., 151, 53–64, https://doi.org/10.1080/00986449608936541, 1996.
Weller, R., Schmidt, K., Teinilä, K., and Hillamo, R.: Natural new particle formation at the coastal Antarctic site Neumayer, Atmos. Chem. Phys., 15, 11399–11410, https://doi.org/10.5194/acp-15-11399-2015, 2015.
Yao, L., Garmash, O., Bianchi, F., Zheng, J., Yan, C., Kontkanen, J., Junninen, H., Mazon, S. B., Ehn, M., Paasonen, P., Sipilä, M., Wang, M., Wang, X., Xiao, S., Chen, H., Lu, Y., Zhang, B., Wang, D., Fu, Q., Geng, F., Li, L., Wang, H., Qiao, L., Yang, X., Chen, J., Kerminen, V.-M., Petäjä, T., Worsnop, D. R., Kulmala, M., and Wang, L.: Atmospheric new particle formation from sulfuric acid and amines in a Chinese megacity, Science, 361, 278–281, https://doi.org/10.1126/science.aao4839, 2018.